Difference between revisions of "Messenger RNA Vaccine"
(130 intermediate revisions by 2 users not shown) | |||
Line 1: | Line 1: | ||
=Roadmap Overview= | Roadmap Creators: [https://www.linkedin.com/in/tomonoshin-aoki/?locale=en_US/ Tomonoshin Aoki], [https://www.linkedin.com/in/wataru-suzuki-743a13233// Wataru Suzuki] | ||
Time Stamp: 4 December 2023 | |||
= Roadmap Overview = | |||
mRNA vaccines represent a breakthrough in immunization technology, offering a novel approach compared to traditional vaccines. Unlike conventional vaccines, which often use weakened or inactive forms of a virus to trigger an immune response, mRNA vaccines work by instructing cells in the body to produce a protein that is part of the virus, leading to an immune response without ever exposing the body to the actual virus. This method allows for quicker development and adaptation, which is particularly advantageous during a rapidly evolving pandemic. The technology's agility enables it to be reprogrammed against new viral strains, providing a significant benefit over traditional vaccines that require longer development times for each new variant. <br> | |||
This roadmap gives a structured overview of the mRNA vaccine technology, breaking down each phase into specific processes and tasks. Each step is crucial to ensuring the safety, efficacy, and availability of the vaccine to the masses. | |||
[[File:Slide2.JPG|frameless|1000px|OPM1]] | |||
=== Vaccine Development Technologies (1VDT) === | |||
* '''2LAV''': Live-attenuated vaccines | |||
* '''2IAV''': Inactivated vaccines | |||
* '''2SUV''': Subunit vaccines | |||
* '''2TXV''': Toxoid vaccines | |||
* '''2VVV''': Viral vector vaccines | |||
* '''2MRV: Messenger RNA (mRNA) vaccine''' | |||
=== Discovery (3DCY) === | |||
The initial phase focuses on identifying and understanding the genetic composition of the target virus and creating a blueprint for the mRNA vaccine. | |||
* '''4SVI''': Structural inference from viral nucleotide information | |||
* '''4MRD''': mRNA design | |||
* '''4MRS''': mRNA Synthesis | |||
* '''4MRM''': mRNA modification | |||
* '''4LMS''': Lipid Membrane Synthesis | |||
=== Development (3DVT) === | |||
This stage involves testing the designed vaccine in human trials and ensuring its safety and efficacy. | |||
* '''4RMH''': Risk Management of the Human Body | |||
* '''4CTM''': Clinical Trial Management | |||
=== Manufacturing Process (3MFP) === | |||
Once approved, the vaccine goes into mass production, ensuring that each component is assembled accurately and in the required quantities. | |||
* '''4MMS''': mRNA mass synthesis (scale-up) | |||
* '''4MLS''': Massive lipid synthesis | |||
* '''4MSB''': Mass synthesis of buffers and other excipients | |||
* '''4MVA''': Mass vaccine assembly | |||
=== Actual Vaccination Situation === | |||
This final stage involves the distribution and administration of the vaccine to individuals, ensuring that they gain immunity. | |||
(Note: No specific sub-processes are provided for this step) | |||
=Design Structure Matrix (DSM) Allocation= | =Design Structure Matrix (DSM) Allocation= | ||
The DSM illustrates the interplay between the tools and methods that constitute the Messenger RNA Vaccine. A 'X' sign denotes that the techniques are interconnected. | The DSM illustrates the interplay between the tools and methods that constitute the Messenger RNA Vaccine. A 'X' sign denotes that the techniques are interconnected.<br> | ||
From the analysis below, we can see that 4MRD(mRNA design) is one of the important technologies because it interacts with many components.<br> | |||
[[File:Images_2.jpg|frameless|700px|DSM showing integration with supporting and enabling technologies]]<br> | |||
=Roadmap Model using OPM= | |||
==Discovery== | |||
The discovery process spans from obtaining the genetic information of the target virus to the phase just before the commencement of human clinical trials.<br> | |||
[[File:2MRV_03_01.jpeg|frameless|600px|OPM1]] | |||
[[File: | ==Development== | ||
The development process begins with the first human clinical trial and continues until the drug receives approval.<br> | |||
[[File:2MRV_03_02.jpeg|frameless|500px|OPM2]] | |||
= | ==Manufacturing process== | ||
The manufacturing process begins after the drug has been approved. It starts with the assembly of each ingredient component and continues through to the shipment of the finished vaccine products.<br> | |||
[[File:2MRV_03_03.jpeg|frameless|500px|OPM3]] | |||
==Actual Vaccination Situation== | |||
The Actual Vaccination Situation refers to the process that starts with receiving the vaccine products at the vaccination site and culminates in individuals gaining immunity after being administered the vaccines.<br> | |||
[[File:2MRV_03_04.jpeg|frameless|1100px|OPM4]] | |||
=Figures of Merit= | =Figures of Merit= | ||
A Figure of Merit has been established as follows.<br> | |||
1. Development period for the novel pathogen: Discovery, Development, and Manufacturing: <br> | |||
These stages represent the timeline of vaccine production, from initial research (discovery), through clinical trials and refinement (development), and finally to large-scale vaccine production (manufacturing). Measuring these in days indicates an emphasis on rapid response capabilities.<br> | |||
2. Time required to respond to mutations: Discovery, Development, and Manufacturing: <br> | |||
Measures how quickly a vaccine can respond to pathogen mutations, as well as development time. The ability to rapidly modify a vaccine in the face of evolving threats is essential to maintaining vaccine efficacy. | |||
Efficacy.<br> | |||
3. Efficacy: Prevent disease/Prevent severity:<br> | |||
This measures a vaccine's effectiveness in preventing disease and its severe consequences in different age groups (children, the elderly, or so on). Percentages indicate the percentage of vaccinated individuals for whom the vaccine effectively prevents disease and severe consequences in each category.<br> | |||
4. Safety: Mild Side Effects/Severe Side Effects:<br> | |||
This aspect assesses the safety profile of a vaccine across different age groups by measuring the incidence of side effects classified as mild or severe. Percentages indicate the percentage of recipients who experienced adverse reactions.<br> | |||
5. Recommended storage temperature<br> | |||
This indicator is critical to the logistic plan as it indicates the temperature range that maintains the stability and efficacy of the vaccine.<br> | |||
6. Ease of injection<br> | |||
This refers to the method of administration (e.g., intramuscular injection or nasal drip) and its convenience. It includes factors such as patient comfort, ease of administration in different settings, and training required for healthcare professionals.<br> | |||
By encompassing these categories, the FOM provides a comprehensive framework for vaccine evaluation. This is especially important for emerging pathogens for which rapid development, adaptability, and effective distribution are key. It also takes into account demographic responses, which are critical for coordinating public health strategies.<br> | |||
[[File:2MRV_04_01.png|frameless|700px|FOM]] | |||
==Vaccine Innovations== | |||
Below is a history of vaccination innovations. While we don't have vaccines for certain diseases, the chart highlights the year in which the causative agent was linked to the disease and the year the vaccine received licensing in the US for various diseases.<br> | |||
[[File:2MRV_04_02.png|left|thumb|500px|'''A timeline of innovation in the development of vaccines'''(Source:https://ourworldindata.org/vaccination)]]<br clear="all"> | |||
Based on the graph above, we have constructed the table below. This table indicates the duration taken for vaccine development. For the years, we've calculated the midpoint between the year the causative agent was linked to the disease and the year the vaccine received licensing in the US.<br> | |||
[[File:2MRV_04_03.png|frameless|1000px|table]]<br> | |||
From the table above, we created the graph below.<br> | |||
[[File:vaccineinnovation.png|frameless|900px|graph]]<br> | |||
In the realm of vaccine development, different vaccines target various pathogens or conditions. It's not accurate to assume that technological advancements will necessarily shorten the development timeframe. A significant portion of the development process is the clinical trial phase, which has become lengthier in recent times. This is due to heightened awareness and stricter requirements for drug safety. Therefore, when assessing technological advancements in this field, we must also consider other Figures of Merit (FOMs) such as the drug's effectiveness and safety profile. However, even taking these into account, progress in drug development isn't always straightforward. External influences, like regulatory standards for medicines and the inherent challenges of targeting diverse diseases, can introduce significant variability in development timelines. | |||
==Vaccine Development for Coronaviruses== | |||
Next, we focused our analysis on the FOM by examining solely the vaccine development for coronaviruses. Specifically, we compared the vaccine development processes for SARS, MERS, and COVID-19(SARS-CoV-2).<br> | |||
===COVID-19=== | |||
[[File:2MRV_04_06.jpg|thumb|500px|'''Milestones and events leading to the successful development of COVID-19 vaccines'''(Source:Bok, K., Sitar, S., Graham, B. S., & Mascola, J. R. (2021). Accelerated COVID-19 vaccine development: milestones, lessons, and prospects. Immunity, 54(8), 1636-1651. )]] | |||
[[File:2MRV_04_05.png|frameless||1000px|COVID-19]]<br clear="left"> | |||
'''Discovery:''' Jan 11, 2020: nCov sequence released. Jan 14: VRC and Moderna Collaborate on COVID-19 vaccine design. Mar 16: 1st Phase 1 trial of USG-supported COVID-19 vaccine begins enrolling (Moderna mRNA).<br> | |||
'''Development:''' Nov 16, Moderna announces results of interim analysis from phase 3 study | |||
===SARS=== | |||
[[File:2MRV_04_07.png|frameless|1000px|SARS]]<br> | |||
SARS was recognized as an emerging infectious disease in March 2003.<br> | |||
'''DNA vaccine:''' the study from December 13, 2004 through May 2, 2005<br> | |||
'''Inactivated SARS-CoV vaccine:''' May to October 2004, 36 healthy people from the Beijing metropolitan area were enrolled into aPhase I clinical trial<br> | |||
===MERS=== | |||
[[File:2MRV_04_08.png|frameless|1000px|MERS]]<br> | |||
MERS was recognized as an emerging infectious disease in September 2012.Only three potential MERS-CoV vaccine candidates have progressed to phase I clinical trials: a DNA vaccine (GLS-5300) and two viral vector-based vaccines (MVA-MERS-S and MERS001).<br> | |||
'''GLS-5300:''' Study Start (Actual) 2016-02.GLS-5300 is a DNA plasmid vaccine that expresses the MERS CoV spike (S) glycoprotein<br> | |||
'''MVA-MERS-S''': Study Start (Actual) 2017-11-28. The vaccine contains a Modified Vaccinia Virus Ankara (MVA) vector expressing the MERS-CoV spike glycoprotein (S).<br> | |||
'''MERS001:''' Study Start (Actual) 2018-03-14<br> | |||
According to the figure above, we created the graph below.<br> | |||
[[File:2MRV_04_09.png|frameless|500px|graph]]<br> | |||
Firstly, the COVID-19 vaccines represent the only successfully developed vaccines against coronaviruses. Their accelerated development can be attributed to prior experiences with SARS and MERS. Interestingly, the development period for the MERS vaccine was longer than that for SARS, and we aim to investigate the reasons behind this extended timeline. One potential explanation could be the challenges associated with the MERS immunological response. The rapid development of COVID-19 vaccines is linked to the research history of SARS and MERS. As illustrated in the figure below, there is a significant similarity among SARS-CoV, MERS-CoV, and SARS-CoV-2. This similarity enabled the developers of the SARS-CoV-2 vaccines to quickly identify the sequence that produces the S protein, which is the primary target of the vaccines.<br> | |||
[[File:2MRV_04_10.jpg|left|thumb|600px|'''The genome and virion structure of coronaviruses (CoVs)''' (Source:Li, Y.-D., Chi, W.-Y., Su, J.-H., Ferrall, L., Hung, C.-F., & Wu, T.-C. (2020). Coronavirus vaccine development: from SARS and MERS to COVID-19. J Biomed Sci, 27(1), 104. )]]<br clear="all"> | |||
=Alignment with Company Strategic Drivers= | =Alignment with Company Strategic Drivers= | ||
{| class="wikitable" | |||
|- | |||
! Number !! Strategic Drivers !! Alignment targets | |||
|- | |||
| 1 || To develop an mRNA vaccine platform that can flexibly address various diseases simply by reconfiguring the nucleotide sequence of mRNA, thereby enhancing manufacturing efficiency in terms of development duration and cost || This roadmap aims for a development period of 300 days or less for any novel pathogen. This driver is currently aligned with the 2MRV technology roadmap''' | |||
|- | |||
| 2 || To make the technical conditions required during transportation easier in order to enable the delivery of the vaccine to a broader range of regions|| This roadmap aims for a recommended storage temperature of 25°C (room temperature). This driver is currently aligned with the 2MRV technology roadmap''' | |||
|- | |||
| 3 || To extend the duration of vaccine efficacy to gain a competitive edge in the market, while considering the balance between re-vaccination frequency and long-term revenue|| This roadmap aims for higher efficacy, but does not set a target for duration of efficacy. This driver is not partially aligned with 2MRV. Also, a longer duration of efficacy may reduce the need for re-vaccination, potentially decreasing long-term revenue opportunities.''' | |||
|} | |||
=Positioning of Company vs. Competition= | =Positioning of Company vs. Competition= | ||
The COVID-19 vaccines being promoted by Pfizer/BioNTech and Moderna are mRNA vaccines. These two companies' vaccines maintain superiority in terms of efficacy compared to others. However, from the perspective of the cold chain, the mRNA vaccines require maintaining a required temperature for maximum shelf life at -70°C and -20°C. This aspect is a challenge for improvement in mRNA vaccines. The cold chain refers to a logistics system that maintains a constant low-temperature state from the time a product is manufactured until it reaches the final consumer. It is especially important for maintaining the quality of temperature-sensitive products such as pharmaceuticals and food. In the case of mRNA vaccines, maintaining their stability requires transportation and storage at extremely low temperatures. | |||
{| class="wikitable" | |||
! Vaccine | |||
! Efficacy | |||
! Type | |||
! mRNA | |||
! Required temperature for maximum shelf life | |||
! State | |||
|- | |||
| <span style="color:red;">Pfizer/BioNTech</span> | |||
| <span style="color:red;">95%</span> | |||
| <span style="color:red;">mRNA</span> | |||
| <span style="color:red;">Y</span> | |||
| <span style="color:red;">-70℃</span> | |||
| <span style="color:red;">Liquid</span> | |||
|- | |||
| AstraZeneca/Oxford | |||
| 70% | |||
| Viral Vector | |||
| N | |||
| 5℃ | |||
| Liquid | |||
|- | |||
| <span style="color:red;">Moderna</span> | |||
| <span style="color:red;">90%</span> | |||
| <span style="color:red;">mRNA</span> | |||
| <span style="color:red;">Y</span> | |||
| <span style="color:red;">-20℃</span> | |||
| <span style="color:red;">Liquid</span> | |||
|- | |||
| Johnson & Johnson | |||
| 66% | |||
| Viral Vector | |||
| N | |||
| 5℃ | |||
| Liquid | |||
|- | |||
| Sinovac | |||
| 66% | |||
| Whole Virus (Inactivated) | |||
| N | |||
| 5℃ | |||
| Liquid | |||
|- | |||
| Gamelaya Institute (Sputnik V) | |||
| 91% | |||
| Viral Vector | |||
| N | |||
| 5℃ | |||
| Liquid | |||
|- | |||
| Sinopharm | |||
| 79% | |||
| Whole Virus (Inactivated) | |||
| N | |||
| 5℃ | |||
| Liquid | |||
|} | |||
[[File:2MRV_7vaccines.png|frameless|600px|graph]]<br> | |||
Caliò, S., Greis, P., & Pfeifer, J. L. (2021). COVID-19 Vaccination: Status Quo. Statista DossierPlus on the COVID-19 Vaccines and the Ongoing Vaccination Campaigns. Statista. Retrieved October 18, 2021, from www.statista.com. | |||
=Technical Model= | =Technical Model= | ||
===Morphological Matrix=== | |||
{| class="wikitable" | |||
! Decision Variable | |||
! 1 | |||
! 2 | |||
! 3 | |||
|- | |||
| '''mRNA Nucleosides Modification''' | |||
| Pseudouridine | |||
| <span style="color:red;">N1-methylpseudouridine</span> | |||
| 5-Methylcytidine | |||
|- | |||
| '''Adjuvant''' | |||
| <span style="color:red;">NO</span> | |||
| Added | |||
| | |||
|- | |||
| '''LNP''' | |||
| <span style="color:red;">Ionizable cationic lipids</span> | |||
| <span style="color:red;">Neutral lipids</span> | |||
| <span style="color:red;">PEGylated lipids</span> | |||
|- | |||
| '''Buffer''' | |||
| <span style="color:red;">Tris-buffered saline</span> | |||
| <span style="color:red;">Phosphate-buffered saline</span> | |||
| <span style="color:red;">HEPES buffer</span> | |||
|- | |||
| '''Vial''' | |||
| <span style="color:red;">Type 1 Borosilicate Glass from Corning/Schott/Nipro</span> | |||
| Valor Glass from Corning | |||
| | |||
|- | |||
| '''Syringe adaptor''' | |||
| <span style="color:red;">NO</span> | |||
| Added | |||
| | |||
|- | |||
| '''Rubber stopper''' | |||
| <span style="color:red;">Silicon</span> | |||
| Teflon (PTFE) | |||
| | |||
|} | |||
<span style="color:red;">※Moderna mRNA-1273 Choice</span> | |||
===Technical Model=== | |||
{| class="wikitable" | |||
! Vaccine | |||
! Efficacy | |||
! Type | |||
! mRNA | |||
! Required temperature for maximum shelf life | |||
! State | |||
|- | |||
| Pfizer/BioNTech | |||
| 95% | |||
| mRNA | |||
| Y | |||
| -70°C | |||
| Liquid | |||
|- | |||
| Pfizer/BioNTech | |||
| 95% | |||
| mRNA | |||
| Y | |||
| 5°C※ | |||
| Solid | |||
|- | |||
| AstraZeneca/Oxford | |||
| 70% | |||
| Viral Vector | |||
| N | |||
| 5°C | |||
| Liquid | |||
|- | |||
| Moderna | |||
| 90% | |||
| mRNA | |||
| Y | |||
| -20°C | |||
| Liquid | |||
|- | |||
| Moderna | |||
| 90% | |||
| mRNA | |||
| Y | |||
| 5°C※ | |||
| Solid | |||
|- | |||
| Johnson & Johnson | |||
| 66% | |||
| Viral Vector | |||
| N | |||
| 5°C | |||
| Liquid | |||
|- | |||
| Sinovac | |||
| 66% | |||
| Whole Virus (Inactivated) | |||
| N | |||
| 5°C | |||
| Liquid | |||
|- | |||
| Gamelaya Institute (Sputnik V) | |||
| 91% | |||
| Viral Vector | |||
| N | |||
| 5°C | |||
| Liquid | |||
|- | |||
| Sinopharm | |||
| 79% | |||
| Whole Virus (Inactivated) | |||
| N | |||
| 5°C | |||
| Liquid | |||
|} | |||
※Kamiya, Mariko, et al. "Stability study of mRNA-lipid nanoparticles exposed to various conditions based on the evaluation between physicochemical properties and their relation with protein expression ability." pharmaceutics 14.11 (2022): 2357. | |||
'''T = Vaccine type (mRNA = -45, non-mRNA = 5) + (only for mRNA) Solid (+50)'''<br> | |||
The model is highly sensitive to both the vaccine type and the state. Both parameters have an equal impact on the temperature, changing it by 50°C. | |||
To visualize this, a tornado chart would have two bars of equal length, one for the vaccine type and one for the state.<br> | |||
[[File:tornade_solid.JPG|frameless|700px|graph]]<br> | |||
<br> | |||
As another FOM, we would like to choose efficacy. <br> | |||
'''Efficacy = β0 + β1 × mRNA'''<br> | |||
Here, | |||
β0(Intercept) = 74.4<br> | |||
β1 (Coefficient for mRNA) = 18.1<br> | |||
[[File:2MRV_Tchart2.png|frameless|600px|graph]]<br> | |||
The formulated model proficiently predicts the optimal temperature to maximize the shelf life of most vaccines. However, discrepancies are observed for Pfizer/BioNTech and Moderna vaccines in their liquid states. The model's sensitivity is notably influenced by the vaccine type and its state. | |||
Regarding efficacy, the incorporation of mRNA appears to enhance vaccine efficacy. Yet, this comes at the cost of reduced temperature stability, presenting a trade-off scenario. | |||
The depth of this analysis is somewhat constrained due to limited data availability. With more extensive data, the model's precision could be significantly improved. | |||
It's crucial to acknowledge the inherent differences across disciplines. For instance, while analytical formulas can be readily developed in fields like aerospace or mechanics, the realm of chemistry and biology operates differently. The governing equation in these domains is the Schrödinger wave equation, which is inherently probabilistic and lacks a definitive analytical solution. Therefore, while formulating models to optimize processes can be beneficial, one must be cognizant of the inherent limitations, especially in the bio-pharma sector. | |||
=Keys Publications and Patent= | |||
==mRNA modification== | |||
===Publications=== | |||
'''Karikó, K., Buckstein, M., Ni, H. and Weissman, D. Suppression of RNA Recognition by Toll-like Receptors: The impact of nucleoside modification and the evolutionary origin of RNA. Immunity 23, 165–175 (2005).'''<br> | |||
First The research by Karikó et al. delves into the role of Toll-like receptors (TLRs) in recognizing RNA molecules and how modifications in RNA nucleosides can impact this recognition. TLRs are a type of protein that plays a crucial role in the innate immune system by detecting foreign molecules, including microbial pathogens. When activated, TLRs can initiate a host's response to infections, triggering potent innate immune responses that lead to subsequent adaptive immune responses. | |||
In the context of vaccine development: | |||
Adjuvants and Vaccine Potency: TLRs are being explored as targets for the development of vaccine adjuvants. Adjuvants are substances added to vaccines to enhance the body's immune response to the vaccine. TLR agonists, which are molecules that can activate TLRs, have been formulated in licensed vaccines to boost their efficacy. For instance, TLR agonists like monophosphoryl lipid A (MPL) and CpG-1018 are used in licensed vaccines for their adjuvant activity. | |||
Reference: Jing-Xing Yang, Jen-Chih Tseng, Guann-Yi Yu, Yunping Luo, Chi-Ying F. Huang, Yi-Ren Hong, Tsung-Hsien Chuang. "Recent Advances in the Development of Toll-like Receptor Agonist-Based Vaccine Adjuvants for Infectious Diseases". | |||
Cancer Immunotherapy: Beyond infectious diseases, TLRs are also being explored in the realm of cancer immunotherapy. The activation of TLRs can promote both innate inflammatory responses and the induction of adaptive immunity, making them potential targets for cancer treatment. Some research has focused on developing nanoparticles that can selectively target endosomal TLRs to potentiate robust cancer vaccination. | |||
Reference: Heming Xia, Mengmeng Qin, Zenghui Wang, Yaoqi Wang, Binlong Chen, Fangjie Wan, Mingmei Tang, Xingquan Pan, Ye Yang, Jianxiong Liu, Ruiyang Zhao, Qiang Zhang, Yiguang Wang. "A pH-/Enzyme-Responsive Nanoparticle Selectively Targets Endosomal Toll-like Receptors to Potentiate Robust Cancer Vaccination". | |||
Selective Immune Response: The study by Karikó et al. suggests that the innate immune system might detect unmodified RNA to selectively respond to bacterial infections or necrotic tissue. This selective response can be harnessed to develop vaccines that elicit a targeted immune reaction against specific pathogens. | |||
In summary, the understanding of TLRs, their activation by RNA, and the impact of nucleoside modifications can provide valuable insights for the development of more effective vaccines and immunotherapies. The work by Karikó and others in this field lays the foundation for innovations in vaccine design and the broader field of immunology. | |||
===Patents=== | |||
Patent Title: '''RNA containing modified nucleosides and methods of use thereof'''<br> | |||
Patent Number: U.S. Patent No. 8,278,036 | |||
Summary: | |||
This patent pertains to the incorporation of modified nucleosides into mRNA. The modification enhances the stability and translational capacity of the mRNA while reducing its immunogenicity. The findings from this patent laid the groundwork for the development of mRNA vaccines, as the modified mRNA is better suited for therapeutic applications due to its enhanced properties. | |||
Importance for Vaccine Development: | |||
Enhanced Stability: The modified mRNA, as described in the patent, has increased stability, making it more suitable for use in vaccines where the mRNA needs to remain intact until it reaches its target cells. | |||
Reduced Immunogenicity: One of the challenges with mRNA-based vaccines is the potential for the mRNA to trigger unwanted immune responses. The modifications described in the patent reduce the immunogenicity of the mRNA, making it safer for use in vaccines. | |||
Increased Translational Capacity: The modified mRNA has a higher capacity for translation, meaning it can produce more of the target protein. This is crucial for mRNA vaccines, where the produced protein acts as an antigen to stimulate an immune response. | |||
Foundation for mRNA Vaccines: The technology and findings from this patent provided a foundation for the development of mRNA-based vaccines, including the COVID-19 vaccines that have been crucial in the global response to the pandemic. | |||
In essence, Katalin Karikó's work on modified mRNA, as described in this patent, played a pivotal role in the development and success of mRNA vaccines. | |||
==Lipid nano-particle== | |||
===Publications=== | |||
'''Reichmuth, Andreas M., et al. "mRNA vaccine delivery using lipid nanoparticles." Therapeutic delivery 7.5 (2016): 319-334.'''<br> | |||
Abstract: Messenger RNA (mRNA) vaccines have the potential to rapidly combat infectious diseases and cancer by delivering one or multiple mRNA molecules that encode disease-specific antigens into cells. Once inside the cells, the mRNA is translated into protein and the specific antigen is presented to the immune system. Lipid nanoparticles (LNPs) are the leading systems for the delivery of mRNA vaccines due to their ability to encapsulate and protect the mRNA from degradation, and facilitate its delivery into cells. This article reviews the composition, physicochemical properties, biocompatibility, and the efficacy of LNPs for mRNA delivery. It also discusses the challenges and future prospects of using LNPs for mRNA vaccine delivery. | |||
Relationship to mRNA Vaccine Development: | |||
The article discusses the potential of mRNA vaccines in combating infectious diseases and cancer. mRNA vaccines work by introducing a piece of mRNA into the body, which instructs cells to produce a protein that triggers an immune response. This immune response can then protect against future infections or diseases. | |||
However, delivering mRNA into cells is challenging because mRNA is unstable and can be easily degraded. This is where lipid nanoparticles (LNPs) come into play. LNPs can encapsulate the mRNA, protecting it from degradation, and help in delivering it into cells where it can be translated into the target protein. | |||
The article reviews the properties of LNPs, such as their composition, physical and chemical properties, and their biocompatibility. It emphasizes that LNPs are the leading delivery systems for mRNA vaccines, highlighting their efficacy in delivering mRNA into cells. | |||
In the context of recent developments, LNPs have been crucial in the successful development and deployment of mRNA-based COVID-19 vaccines, such as those developed by Pfizer-BioNTech and Moderna. These vaccines use LNPs to deliver the mRNA encoding the spike protein of the SARS-CoV-2 virus, triggering an immune response against the virus. | |||
In summary, the article underscores the importance of LNPs in the development of mRNA vaccines, detailing their role in ensuring the stability and efficient delivery of mRNA into cells, which is pivotal for the vaccine's effectiveness. | |||
===Patents=== | |||
Title: '''Lipid nanoparticle formulations'''<br> | |||
Assignee: Acuitas Therapeutics Inc.<br> | |||
Inventors: Michael J. Hope, Barbara Mui, Paulo Jia Ching Lin, Christopher J. Barbosa, Thomas D. Madden, Steven M. Ansell, Xinyao Du<br> | |||
Patent Number: US11712481B2<br> | |||
Status: Active<br> | |||
Anticipated Expiration: 2036-10-28<br> | |||
Abstract: The patent describes improved formulations of lipid nanoparticles. These nanoparticles can be used for the delivery of therapeutic agents. The methods for preparing these lipid nanoparticles are also disclosed. | |||
Key Features/Claims: | |||
Composition of the Lipid Nanoparticle (LNP): | |||
Contains 47 to 48 mol percent of a specific cationic lipid. | |||
Contains a neutral lipid. | |||
Contains a steroid, with cholesterol being a noted example. | |||
Contains a polymer conjugated lipid, wherein a pegylated lipid is an example. | |||
Encapsulates or associates with a nucleic acid, including antisense and messenger RNA (mRNA). | |||
Structural Details of the Cationic Lipid: | |||
Detailed molecular structures of the cationic lipid are provided, specifying potential functional groups, bond types, and ranges for carbon chain lengths. | |||
Usage: | |||
The LNP can be used to administer a therapeutic agent to a patient. | |||
It can be used to treat diseases where the therapeutic agent within the LNP is effective against that disease. | |||
Other Features: | |||
Some claims specify the preferred concentrations or molar ratios of the components within the LNP. For instance, the steroid could be present in a concentration ranging from 32 to 40 mol percent. | |||
Relevance for mRNA Vaccine Development: | |||
mRNA vaccines require a delivery system to effectively enter human cells without being degraded. The lipid nanoparticles described in this patent can serve as that delivery system. Specifically: | |||
mRNA Encapsulation: The LNP described encapsulates or associates with nucleic acids, which includes mRNA. By doing so, it can protect the mRNA from degradation and aid in its delivery into cells. | |||
Targeted Delivery: The specific formulation and characteristics of the LNP could potentially ensure that the mRNA is delivered more efficiently to the target cells. | |||
Improved Formulation: The patent suggests that these are improved formulations, which might imply better stability, safety, or efficacy compared to previous LNPs. | |||
Overall, this patent could represent an advancement in the delivery mechanisms for mRNA vaccines, which is crucial for the effectiveness of the vaccine. | |||
=List of R&D project= | |||
===Project 1: Development of Freeze-Drying Technology for mRNA Vaccines=== | |||
Objective: To enhance the stability of mRNA vaccines through freeze-drying technology, enabling storage at room temperatures and reducing dependence on cold chain logistics. | |||
Description: mRNA vaccines currently require storage at extremely low temperatures (-20 to -70 degrees Celsius), posing challenges in distribution, especially in low- and middle-income countries (LMICs) where maintaining such cold chains is difficult. This project aims to develop freeze-drying technology that allows mRNA vaccines to be stored at up to 37 degrees Celsius for at least 12 weeks, significantly improving their stability and accessibility. | |||
Reference Study: Meulewaeter, Sofie, et al. "Continuous freeze-drying of messenger RNA lipid nanoparticles enables storage at higher temperatures." Journal of Controlled Release 357 (2023): 149-160. | |||
===Project 2: Development of Inhalable mRNA Vaccines=== | |||
Objective: To increase the effectiveness and ease of administration of mRNA vaccines by developing an inhalable form, enhancing immune response at the initial site of pathogen entry. | |||
Description: This project focuses on creating inhalable mRNA vaccines to potentially enhance immune responses more effectively than injectable forms. Inhalable vaccines can be more appealing to those hesitant about needles and may offer prolonged effectiveness. The project will explore formulations and delivery mechanisms suitable for respiratory administration. | |||
Reference Study: Roh, Esther H., Catherine A. Fromen, and Millicent O. Sullivan. "Inhalable mRNA vaccines for respiratory diseases: a roadmap." Current Opinion in Biotechnology 74 (2022): 104-109. | |||
===Project 3: Continuous Manufacturing Process for mRNA Vaccines=== | |||
Objective: To optimize mRNA vaccine production by implementing continuous manufacturing processes, reducing costs, and improving efficiency. | |||
Description: This project aims to address the bottlenecks in vaccine supply, particularly in manufacturing capacity and resource utilization. By adopting continuous manufacturing processes, complemented by quality-by-design principles, digital twins, process analytical technology, and control automation strategies, the project seeks to enhance production efficiency. This approach is expected to reduce costs significantly, streamline personnel training, optimize resource use, and accelerate product release. | |||
Expected Outcome: A potential cost reduction by a factor of 5 (from EUR 0.380 per dose to EUR 0.085 per dose), mainly due to reduced personnel and consumable needs, and a decrease in batch failures and out-of-specification products. | |||
Reference Study: Schmidt, Axel, et al. "Process Automation and Control Strategy by Quality-by-Design in Total Continuous mRNA Manufacturing Platforms." Processes 10.9 (2022): 1783. | |||
=Financial Model= | =Financial Model= | ||
[[File:2MRV_FM_Table.png|frameless|1200px|graph]]<br> | |||
[[File:2MRV_FM_Graph.png|frameless|1500px|graph]]<br> | |||
=Technology Strategy Statement= | |||
Our strategic vision in mRNA vaccine technology is centered on rapidly developing and scaling up highly effective and stable vaccines at an affordable cost. By pioneering the Continuous Manufacturing Process for mRNA Vaccines, we aim to significantly reduce vaccine costs, streamline the manufacturing process, and enhance the responsiveness of the supply chain. This approach not only optimizes production efficiency but also aligns with our commitment to making life-saving vaccines more accessible globally. | |||
In parallel, our focus on the Development of Inhalable mRNA Vaccines is set to broaden vaccine accessibility and acceptance. This innovative form of vaccine administration is anticipated to increase vaccination rates, particularly in populations that are hesitant about traditional injection methods. The inhalable vaccine represents a leap forward in improving administration and efficacy, offering a more user-friendly and potentially more effective alternative. | |||
Lastly, our development of Freeze-Drying Technology for mRNA Vaccines is a critical component of our strategy. This technology is poised to revolutionize the distribution of vaccines, especially in low- and middle-income countries (LMICs), by significantly reducing the reliance on cold chain logistics. This advancement will facilitate wider distribution and ensure that vaccines remain stable and effective, even in challenging environments. | |||
Together, these initiatives form the cornerstone of our technology strategy, representing a comprehensive approach to overcoming current barriers in vaccine distribution and administration. Our strategy is visualized in a “swoosh” chart, which illustrates the dynamic progression of these projects from conception through development, growth, and eventual widespread implementation. This chart symbolizes our journey towards creating more accessible, efficient, and effective vaccine solutions for the world. | |||
Adaptive R&D Strategy: Recognizing the variability of financial landscapes, we have a flexible R&D plan. While our optimal scenario involves parallel development of all three projects, we can adapt to more linear progression if financial constraints arise. In this model, we would sequentially initiate each project, with each one's revenue funding the next. This staged approach ensures financial viability and continuous innovation, even in fluctuating economic conditions. | |||
[[File:2MRV_Swoosh.png|frameless|800px|graph]]<br> |
Latest revision as of 22:37, 4 December 2023
Roadmap Creators: Tomonoshin Aoki, Wataru Suzuki
Time Stamp: 4 December 2023
Roadmap Overview
mRNA vaccines represent a breakthrough in immunization technology, offering a novel approach compared to traditional vaccines. Unlike conventional vaccines, which often use weakened or inactive forms of a virus to trigger an immune response, mRNA vaccines work by instructing cells in the body to produce a protein that is part of the virus, leading to an immune response without ever exposing the body to the actual virus. This method allows for quicker development and adaptation, which is particularly advantageous during a rapidly evolving pandemic. The technology's agility enables it to be reprogrammed against new viral strains, providing a significant benefit over traditional vaccines that require longer development times for each new variant.
This roadmap gives a structured overview of the mRNA vaccine technology, breaking down each phase into specific processes and tasks. Each step is crucial to ensuring the safety, efficacy, and availability of the vaccine to the masses.
Vaccine Development Technologies (1VDT)
- 2LAV: Live-attenuated vaccines
- 2IAV: Inactivated vaccines
- 2SUV: Subunit vaccines
- 2TXV: Toxoid vaccines
- 2VVV: Viral vector vaccines
- 2MRV: Messenger RNA (mRNA) vaccine
Discovery (3DCY)
The initial phase focuses on identifying and understanding the genetic composition of the target virus and creating a blueprint for the mRNA vaccine.
- 4SVI: Structural inference from viral nucleotide information
- 4MRD: mRNA design
- 4MRS: mRNA Synthesis
- 4MRM: mRNA modification
- 4LMS: Lipid Membrane Synthesis
Development (3DVT)
This stage involves testing the designed vaccine in human trials and ensuring its safety and efficacy.
- 4RMH: Risk Management of the Human Body
- 4CTM: Clinical Trial Management
Manufacturing Process (3MFP)
Once approved, the vaccine goes into mass production, ensuring that each component is assembled accurately and in the required quantities.
- 4MMS: mRNA mass synthesis (scale-up)
- 4MLS: Massive lipid synthesis
- 4MSB: Mass synthesis of buffers and other excipients
- 4MVA: Mass vaccine assembly
Actual Vaccination Situation
This final stage involves the distribution and administration of the vaccine to individuals, ensuring that they gain immunity. (Note: No specific sub-processes are provided for this step)
Design Structure Matrix (DSM) Allocation
The DSM illustrates the interplay between the tools and methods that constitute the Messenger RNA Vaccine. A 'X' sign denotes that the techniques are interconnected.
From the analysis below, we can see that 4MRD(mRNA design) is one of the important technologies because it interacts with many components.
Roadmap Model using OPM
Discovery
The discovery process spans from obtaining the genetic information of the target virus to the phase just before the commencement of human clinical trials.
Development
The development process begins with the first human clinical trial and continues until the drug receives approval.
Manufacturing process
The manufacturing process begins after the drug has been approved. It starts with the assembly of each ingredient component and continues through to the shipment of the finished vaccine products.
Actual Vaccination Situation
The Actual Vaccination Situation refers to the process that starts with receiving the vaccine products at the vaccination site and culminates in individuals gaining immunity after being administered the vaccines.
Figures of Merit
A Figure of Merit has been established as follows.
1. Development period for the novel pathogen: Discovery, Development, and Manufacturing:
These stages represent the timeline of vaccine production, from initial research (discovery), through clinical trials and refinement (development), and finally to large-scale vaccine production (manufacturing). Measuring these in days indicates an emphasis on rapid response capabilities.
2. Time required to respond to mutations: Discovery, Development, and Manufacturing:
Measures how quickly a vaccine can respond to pathogen mutations, as well as development time. The ability to rapidly modify a vaccine in the face of evolving threats is essential to maintaining vaccine efficacy.
Efficacy.
3. Efficacy: Prevent disease/Prevent severity:
This measures a vaccine's effectiveness in preventing disease and its severe consequences in different age groups (children, the elderly, or so on). Percentages indicate the percentage of vaccinated individuals for whom the vaccine effectively prevents disease and severe consequences in each category.
4. Safety: Mild Side Effects/Severe Side Effects:
This aspect assesses the safety profile of a vaccine across different age groups by measuring the incidence of side effects classified as mild or severe. Percentages indicate the percentage of recipients who experienced adverse reactions.
5. Recommended storage temperature
This indicator is critical to the logistic plan as it indicates the temperature range that maintains the stability and efficacy of the vaccine.
6. Ease of injection
This refers to the method of administration (e.g., intramuscular injection or nasal drip) and its convenience. It includes factors such as patient comfort, ease of administration in different settings, and training required for healthcare professionals.
By encompassing these categories, the FOM provides a comprehensive framework for vaccine evaluation. This is especially important for emerging pathogens for which rapid development, adaptability, and effective distribution are key. It also takes into account demographic responses, which are critical for coordinating public health strategies.
Vaccine Innovations
Below is a history of vaccination innovations. While we don't have vaccines for certain diseases, the chart highlights the year in which the causative agent was linked to the disease and the year the vaccine received licensing in the US for various diseases.
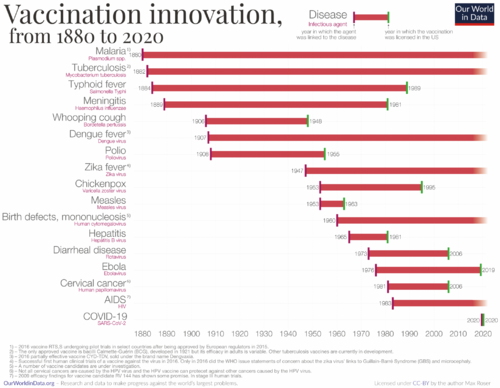
Based on the graph above, we have constructed the table below. This table indicates the duration taken for vaccine development. For the years, we've calculated the midpoint between the year the causative agent was linked to the disease and the year the vaccine received licensing in the US.
From the table above, we created the graph below.
In the realm of vaccine development, different vaccines target various pathogens or conditions. It's not accurate to assume that technological advancements will necessarily shorten the development timeframe. A significant portion of the development process is the clinical trial phase, which has become lengthier in recent times. This is due to heightened awareness and stricter requirements for drug safety. Therefore, when assessing technological advancements in this field, we must also consider other Figures of Merit (FOMs) such as the drug's effectiveness and safety profile. However, even taking these into account, progress in drug development isn't always straightforward. External influences, like regulatory standards for medicines and the inherent challenges of targeting diverse diseases, can introduce significant variability in development timelines.
Next, we focused our analysis on the FOM by examining solely the vaccine development for coronaviruses. Specifically, we compared the vaccine development processes for SARS, MERS, and COVID-19(SARS-CoV-2).
COVID-19
Discovery: Jan 11, 2020: nCov sequence released. Jan 14: VRC and Moderna Collaborate on COVID-19 vaccine design. Mar 16: 1st Phase 1 trial of USG-supported COVID-19 vaccine begins enrolling (Moderna mRNA).
Development: Nov 16, Moderna announces results of interim analysis from phase 3 study
SARS
SARS was recognized as an emerging infectious disease in March 2003.
DNA vaccine: the study from December 13, 2004 through May 2, 2005
Inactivated SARS-CoV vaccine: May to October 2004, 36 healthy people from the Beijing metropolitan area were enrolled into aPhase I clinical trial
MERS
MERS was recognized as an emerging infectious disease in September 2012.Only three potential MERS-CoV vaccine candidates have progressed to phase I clinical trials: a DNA vaccine (GLS-5300) and two viral vector-based vaccines (MVA-MERS-S and MERS001).
GLS-5300: Study Start (Actual) 2016-02.GLS-5300 is a DNA plasmid vaccine that expresses the MERS CoV spike (S) glycoprotein
MVA-MERS-S: Study Start (Actual) 2017-11-28. The vaccine contains a Modified Vaccinia Virus Ankara (MVA) vector expressing the MERS-CoV spike glycoprotein (S).
MERS001: Study Start (Actual) 2018-03-14
According to the figure above, we created the graph below.
Firstly, the COVID-19 vaccines represent the only successfully developed vaccines against coronaviruses. Their accelerated development can be attributed to prior experiences with SARS and MERS. Interestingly, the development period for the MERS vaccine was longer than that for SARS, and we aim to investigate the reasons behind this extended timeline. One potential explanation could be the challenges associated with the MERS immunological response. The rapid development of COVID-19 vaccines is linked to the research history of SARS and MERS. As illustrated in the figure below, there is a significant similarity among SARS-CoV, MERS-CoV, and SARS-CoV-2. This similarity enabled the developers of the SARS-CoV-2 vaccines to quickly identify the sequence that produces the S protein, which is the primary target of the vaccines.
Alignment with Company Strategic Drivers
Number | Strategic Drivers | Alignment targets |
---|---|---|
1 | To develop an mRNA vaccine platform that can flexibly address various diseases simply by reconfiguring the nucleotide sequence of mRNA, thereby enhancing manufacturing efficiency in terms of development duration and cost | This roadmap aims for a development period of 300 days or less for any novel pathogen. This driver is currently aligned with the 2MRV technology roadmap |
2 | To make the technical conditions required during transportation easier in order to enable the delivery of the vaccine to a broader range of regions | This roadmap aims for a recommended storage temperature of 25°C (room temperature). This driver is currently aligned with the 2MRV technology roadmap |
3 | To extend the duration of vaccine efficacy to gain a competitive edge in the market, while considering the balance between re-vaccination frequency and long-term revenue | This roadmap aims for higher efficacy, but does not set a target for duration of efficacy. This driver is not partially aligned with 2MRV. Also, a longer duration of efficacy may reduce the need for re-vaccination, potentially decreasing long-term revenue opportunities. |
Positioning of Company vs. Competition
The COVID-19 vaccines being promoted by Pfizer/BioNTech and Moderna are mRNA vaccines. These two companies' vaccines maintain superiority in terms of efficacy compared to others. However, from the perspective of the cold chain, the mRNA vaccines require maintaining a required temperature for maximum shelf life at -70°C and -20°C. This aspect is a challenge for improvement in mRNA vaccines. The cold chain refers to a logistics system that maintains a constant low-temperature state from the time a product is manufactured until it reaches the final consumer. It is especially important for maintaining the quality of temperature-sensitive products such as pharmaceuticals and food. In the case of mRNA vaccines, maintaining their stability requires transportation and storage at extremely low temperatures.
Vaccine | Efficacy | Type | mRNA | Required temperature for maximum shelf life | State |
---|---|---|---|---|---|
Pfizer/BioNTech | 95% | mRNA | Y | -70℃ | Liquid |
AstraZeneca/Oxford | 70% | Viral Vector | N | 5℃ | Liquid |
Moderna | 90% | mRNA | Y | -20℃ | Liquid |
Johnson & Johnson | 66% | Viral Vector | N | 5℃ | Liquid |
Sinovac | 66% | Whole Virus (Inactivated) | N | 5℃ | Liquid |
Gamelaya Institute (Sputnik V) | 91% | Viral Vector | N | 5℃ | Liquid |
Sinopharm | 79% | Whole Virus (Inactivated) | N | 5℃ | Liquid |
Caliò, S., Greis, P., & Pfeifer, J. L. (2021). COVID-19 Vaccination: Status Quo. Statista DossierPlus on the COVID-19 Vaccines and the Ongoing Vaccination Campaigns. Statista. Retrieved October 18, 2021, from www.statista.com.
Technical Model
Morphological Matrix
Decision Variable | 1 | 2 | 3 |
---|---|---|---|
mRNA Nucleosides Modification | Pseudouridine | N1-methylpseudouridine | 5-Methylcytidine |
Adjuvant | NO | Added | |
LNP | Ionizable cationic lipids | Neutral lipids | PEGylated lipids |
Buffer | Tris-buffered saline | Phosphate-buffered saline | HEPES buffer |
Vial | Type 1 Borosilicate Glass from Corning/Schott/Nipro | Valor Glass from Corning | |
Syringe adaptor | NO | Added | |
Rubber stopper | Silicon | Teflon (PTFE) |
※Moderna mRNA-1273 Choice
Technical Model
Vaccine | Efficacy | Type | mRNA | Required temperature for maximum shelf life | State |
---|---|---|---|---|---|
Pfizer/BioNTech | 95% | mRNA | Y | -70°C | Liquid |
Pfizer/BioNTech | 95% | mRNA | Y | 5°C※ | Solid |
AstraZeneca/Oxford | 70% | Viral Vector | N | 5°C | Liquid |
Moderna | 90% | mRNA | Y | -20°C | Liquid |
Moderna | 90% | mRNA | Y | 5°C※ | Solid |
Johnson & Johnson | 66% | Viral Vector | N | 5°C | Liquid |
Sinovac | 66% | Whole Virus (Inactivated) | N | 5°C | Liquid |
Gamelaya Institute (Sputnik V) | 91% | Viral Vector | N | 5°C | Liquid |
Sinopharm | 79% | Whole Virus (Inactivated) | N | 5°C | Liquid |
※Kamiya, Mariko, et al. "Stability study of mRNA-lipid nanoparticles exposed to various conditions based on the evaluation between physicochemical properties and their relation with protein expression ability." pharmaceutics 14.11 (2022): 2357.
T = Vaccine type (mRNA = -45, non-mRNA = 5) + (only for mRNA) Solid (+50)
The model is highly sensitive to both the vaccine type and the state. Both parameters have an equal impact on the temperature, changing it by 50°C.
To visualize this, a tornado chart would have two bars of equal length, one for the vaccine type and one for the state.
As another FOM, we would like to choose efficacy.
Efficacy = β0 + β1 × mRNA
Here,
β0(Intercept) = 74.4
β1 (Coefficient for mRNA) = 18.1
The formulated model proficiently predicts the optimal temperature to maximize the shelf life of most vaccines. However, discrepancies are observed for Pfizer/BioNTech and Moderna vaccines in their liquid states. The model's sensitivity is notably influenced by the vaccine type and its state.
Regarding efficacy, the incorporation of mRNA appears to enhance vaccine efficacy. Yet, this comes at the cost of reduced temperature stability, presenting a trade-off scenario.
The depth of this analysis is somewhat constrained due to limited data availability. With more extensive data, the model's precision could be significantly improved.
It's crucial to acknowledge the inherent differences across disciplines. For instance, while analytical formulas can be readily developed in fields like aerospace or mechanics, the realm of chemistry and biology operates differently. The governing equation in these domains is the Schrödinger wave equation, which is inherently probabilistic and lacks a definitive analytical solution. Therefore, while formulating models to optimize processes can be beneficial, one must be cognizant of the inherent limitations, especially in the bio-pharma sector.
Keys Publications and Patent
mRNA modification
Publications
Karikó, K., Buckstein, M., Ni, H. and Weissman, D. Suppression of RNA Recognition by Toll-like Receptors: The impact of nucleoside modification and the evolutionary origin of RNA. Immunity 23, 165–175 (2005).
First The research by Karikó et al. delves into the role of Toll-like receptors (TLRs) in recognizing RNA molecules and how modifications in RNA nucleosides can impact this recognition. TLRs are a type of protein that plays a crucial role in the innate immune system by detecting foreign molecules, including microbial pathogens. When activated, TLRs can initiate a host's response to infections, triggering potent innate immune responses that lead to subsequent adaptive immune responses.
In the context of vaccine development:
Adjuvants and Vaccine Potency: TLRs are being explored as targets for the development of vaccine adjuvants. Adjuvants are substances added to vaccines to enhance the body's immune response to the vaccine. TLR agonists, which are molecules that can activate TLRs, have been formulated in licensed vaccines to boost their efficacy. For instance, TLR agonists like monophosphoryl lipid A (MPL) and CpG-1018 are used in licensed vaccines for their adjuvant activity. Reference: Jing-Xing Yang, Jen-Chih Tseng, Guann-Yi Yu, Yunping Luo, Chi-Ying F. Huang, Yi-Ren Hong, Tsung-Hsien Chuang. "Recent Advances in the Development of Toll-like Receptor Agonist-Based Vaccine Adjuvants for Infectious Diseases".
Cancer Immunotherapy: Beyond infectious diseases, TLRs are also being explored in the realm of cancer immunotherapy. The activation of TLRs can promote both innate inflammatory responses and the induction of adaptive immunity, making them potential targets for cancer treatment. Some research has focused on developing nanoparticles that can selectively target endosomal TLRs to potentiate robust cancer vaccination. Reference: Heming Xia, Mengmeng Qin, Zenghui Wang, Yaoqi Wang, Binlong Chen, Fangjie Wan, Mingmei Tang, Xingquan Pan, Ye Yang, Jianxiong Liu, Ruiyang Zhao, Qiang Zhang, Yiguang Wang. "A pH-/Enzyme-Responsive Nanoparticle Selectively Targets Endosomal Toll-like Receptors to Potentiate Robust Cancer Vaccination".
Selective Immune Response: The study by Karikó et al. suggests that the innate immune system might detect unmodified RNA to selectively respond to bacterial infections or necrotic tissue. This selective response can be harnessed to develop vaccines that elicit a targeted immune reaction against specific pathogens.
In summary, the understanding of TLRs, their activation by RNA, and the impact of nucleoside modifications can provide valuable insights for the development of more effective vaccines and immunotherapies. The work by Karikó and others in this field lays the foundation for innovations in vaccine design and the broader field of immunology.
Patents
Patent Title: RNA containing modified nucleosides and methods of use thereof
Patent Number: U.S. Patent No. 8,278,036
Summary: This patent pertains to the incorporation of modified nucleosides into mRNA. The modification enhances the stability and translational capacity of the mRNA while reducing its immunogenicity. The findings from this patent laid the groundwork for the development of mRNA vaccines, as the modified mRNA is better suited for therapeutic applications due to its enhanced properties.
Importance for Vaccine Development:
Enhanced Stability: The modified mRNA, as described in the patent, has increased stability, making it more suitable for use in vaccines where the mRNA needs to remain intact until it reaches its target cells.
Reduced Immunogenicity: One of the challenges with mRNA-based vaccines is the potential for the mRNA to trigger unwanted immune responses. The modifications described in the patent reduce the immunogenicity of the mRNA, making it safer for use in vaccines.
Increased Translational Capacity: The modified mRNA has a higher capacity for translation, meaning it can produce more of the target protein. This is crucial for mRNA vaccines, where the produced protein acts as an antigen to stimulate an immune response.
Foundation for mRNA Vaccines: The technology and findings from this patent provided a foundation for the development of mRNA-based vaccines, including the COVID-19 vaccines that have been crucial in the global response to the pandemic.
In essence, Katalin Karikó's work on modified mRNA, as described in this patent, played a pivotal role in the development and success of mRNA vaccines.
Lipid nano-particle
Publications
Reichmuth, Andreas M., et al. "mRNA vaccine delivery using lipid nanoparticles." Therapeutic delivery 7.5 (2016): 319-334.
Abstract: Messenger RNA (mRNA) vaccines have the potential to rapidly combat infectious diseases and cancer by delivering one or multiple mRNA molecules that encode disease-specific antigens into cells. Once inside the cells, the mRNA is translated into protein and the specific antigen is presented to the immune system. Lipid nanoparticles (LNPs) are the leading systems for the delivery of mRNA vaccines due to their ability to encapsulate and protect the mRNA from degradation, and facilitate its delivery into cells. This article reviews the composition, physicochemical properties, biocompatibility, and the efficacy of LNPs for mRNA delivery. It also discusses the challenges and future prospects of using LNPs for mRNA vaccine delivery.
Relationship to mRNA Vaccine Development: The article discusses the potential of mRNA vaccines in combating infectious diseases and cancer. mRNA vaccines work by introducing a piece of mRNA into the body, which instructs cells to produce a protein that triggers an immune response. This immune response can then protect against future infections or diseases.
However, delivering mRNA into cells is challenging because mRNA is unstable and can be easily degraded. This is where lipid nanoparticles (LNPs) come into play. LNPs can encapsulate the mRNA, protecting it from degradation, and help in delivering it into cells where it can be translated into the target protein.
The article reviews the properties of LNPs, such as their composition, physical and chemical properties, and their biocompatibility. It emphasizes that LNPs are the leading delivery systems for mRNA vaccines, highlighting their efficacy in delivering mRNA into cells.
In the context of recent developments, LNPs have been crucial in the successful development and deployment of mRNA-based COVID-19 vaccines, such as those developed by Pfizer-BioNTech and Moderna. These vaccines use LNPs to deliver the mRNA encoding the spike protein of the SARS-CoV-2 virus, triggering an immune response against the virus.
In summary, the article underscores the importance of LNPs in the development of mRNA vaccines, detailing their role in ensuring the stability and efficient delivery of mRNA into cells, which is pivotal for the vaccine's effectiveness.
Patents
Title: Lipid nanoparticle formulations
Assignee: Acuitas Therapeutics Inc.
Inventors: Michael J. Hope, Barbara Mui, Paulo Jia Ching Lin, Christopher J. Barbosa, Thomas D. Madden, Steven M. Ansell, Xinyao Du
Patent Number: US11712481B2
Status: Active
Anticipated Expiration: 2036-10-28
Abstract: The patent describes improved formulations of lipid nanoparticles. These nanoparticles can be used for the delivery of therapeutic agents. The methods for preparing these lipid nanoparticles are also disclosed.
Key Features/Claims: Composition of the Lipid Nanoparticle (LNP): Contains 47 to 48 mol percent of a specific cationic lipid. Contains a neutral lipid. Contains a steroid, with cholesterol being a noted example. Contains a polymer conjugated lipid, wherein a pegylated lipid is an example. Encapsulates or associates with a nucleic acid, including antisense and messenger RNA (mRNA). Structural Details of the Cationic Lipid: Detailed molecular structures of the cationic lipid are provided, specifying potential functional groups, bond types, and ranges for carbon chain lengths. Usage: The LNP can be used to administer a therapeutic agent to a patient. It can be used to treat diseases where the therapeutic agent within the LNP is effective against that disease. Other Features: Some claims specify the preferred concentrations or molar ratios of the components within the LNP. For instance, the steroid could be present in a concentration ranging from 32 to 40 mol percent.
Relevance for mRNA Vaccine Development: mRNA vaccines require a delivery system to effectively enter human cells without being degraded. The lipid nanoparticles described in this patent can serve as that delivery system. Specifically: mRNA Encapsulation: The LNP described encapsulates or associates with nucleic acids, which includes mRNA. By doing so, it can protect the mRNA from degradation and aid in its delivery into cells. Targeted Delivery: The specific formulation and characteristics of the LNP could potentially ensure that the mRNA is delivered more efficiently to the target cells. Improved Formulation: The patent suggests that these are improved formulations, which might imply better stability, safety, or efficacy compared to previous LNPs. Overall, this patent could represent an advancement in the delivery mechanisms for mRNA vaccines, which is crucial for the effectiveness of the vaccine.
List of R&D project
Project 1: Development of Freeze-Drying Technology for mRNA Vaccines
Objective: To enhance the stability of mRNA vaccines through freeze-drying technology, enabling storage at room temperatures and reducing dependence on cold chain logistics.
Description: mRNA vaccines currently require storage at extremely low temperatures (-20 to -70 degrees Celsius), posing challenges in distribution, especially in low- and middle-income countries (LMICs) where maintaining such cold chains is difficult. This project aims to develop freeze-drying technology that allows mRNA vaccines to be stored at up to 37 degrees Celsius for at least 12 weeks, significantly improving their stability and accessibility.
Reference Study: Meulewaeter, Sofie, et al. "Continuous freeze-drying of messenger RNA lipid nanoparticles enables storage at higher temperatures." Journal of Controlled Release 357 (2023): 149-160.
Project 2: Development of Inhalable mRNA Vaccines
Objective: To increase the effectiveness and ease of administration of mRNA vaccines by developing an inhalable form, enhancing immune response at the initial site of pathogen entry.
Description: This project focuses on creating inhalable mRNA vaccines to potentially enhance immune responses more effectively than injectable forms. Inhalable vaccines can be more appealing to those hesitant about needles and may offer prolonged effectiveness. The project will explore formulations and delivery mechanisms suitable for respiratory administration.
Reference Study: Roh, Esther H., Catherine A. Fromen, and Millicent O. Sullivan. "Inhalable mRNA vaccines for respiratory diseases: a roadmap." Current Opinion in Biotechnology 74 (2022): 104-109.
Project 3: Continuous Manufacturing Process for mRNA Vaccines
Objective: To optimize mRNA vaccine production by implementing continuous manufacturing processes, reducing costs, and improving efficiency.
Description: This project aims to address the bottlenecks in vaccine supply, particularly in manufacturing capacity and resource utilization. By adopting continuous manufacturing processes, complemented by quality-by-design principles, digital twins, process analytical technology, and control automation strategies, the project seeks to enhance production efficiency. This approach is expected to reduce costs significantly, streamline personnel training, optimize resource use, and accelerate product release.
Expected Outcome: A potential cost reduction by a factor of 5 (from EUR 0.380 per dose to EUR 0.085 per dose), mainly due to reduced personnel and consumable needs, and a decrease in batch failures and out-of-specification products.
Reference Study: Schmidt, Axel, et al. "Process Automation and Control Strategy by Quality-by-Design in Total Continuous mRNA Manufacturing Platforms." Processes 10.9 (2022): 1783.
Financial Model
Technology Strategy Statement
Our strategic vision in mRNA vaccine technology is centered on rapidly developing and scaling up highly effective and stable vaccines at an affordable cost. By pioneering the Continuous Manufacturing Process for mRNA Vaccines, we aim to significantly reduce vaccine costs, streamline the manufacturing process, and enhance the responsiveness of the supply chain. This approach not only optimizes production efficiency but also aligns with our commitment to making life-saving vaccines more accessible globally.
In parallel, our focus on the Development of Inhalable mRNA Vaccines is set to broaden vaccine accessibility and acceptance. This innovative form of vaccine administration is anticipated to increase vaccination rates, particularly in populations that are hesitant about traditional injection methods. The inhalable vaccine represents a leap forward in improving administration and efficacy, offering a more user-friendly and potentially more effective alternative.
Lastly, our development of Freeze-Drying Technology for mRNA Vaccines is a critical component of our strategy. This technology is poised to revolutionize the distribution of vaccines, especially in low- and middle-income countries (LMICs), by significantly reducing the reliance on cold chain logistics. This advancement will facilitate wider distribution and ensure that vaccines remain stable and effective, even in challenging environments.
Together, these initiatives form the cornerstone of our technology strategy, representing a comprehensive approach to overcoming current barriers in vaccine distribution and administration. Our strategy is visualized in a “swoosh” chart, which illustrates the dynamic progression of these projects from conception through development, growth, and eventual widespread implementation. This chart symbolizes our journey towards creating more accessible, efficient, and effective vaccine solutions for the world.
Adaptive R&D Strategy: Recognizing the variability of financial landscapes, we have a flexible R&D plan. While our optimal scenario involves parallel development of all three projects, we can adapt to more linear progression if financial constraints arise. In this model, we would sequentially initiate each project, with each one's revenue funding the next. This staged approach ensures financial viability and continuous innovation, even in fluctuating economic conditions.