Difference between revisions of "Superconducting Cable"
(44 intermediate revisions by 2 users not shown) | |||
Line 1: | Line 1: | ||
Time Stamp: 5 December 2023 | |||
=Technology Roadmap Sections and Deliverables= | =Technology Roadmap Sections and Deliverables= | ||
Line 5: | Line 7: | ||
==Roadmap Overview== | ==Roadmap Overview== | ||
[[File: 3SC_Section1_1.png| | [[File: 3SC_Section1_1.png|500px|thumb|right|Feature of Superconductivity Phenomenon]][[File: 3SC_Section1_2.jpg|500px|thumb|right|Typical HTS Cable Structure <small>(https://eeecommunity.blogspot.com/2016/09/typical-high-temperature-superconductor.html)</small>]]The feature of the superconductivity phenomenon, the basis of this roadmap technology (3SC - Superconducting Cable), is an unusual property of certain substances, including metals, alloys, and ceramics, in which electrical resistance drops to zero when the temperature is reduced below a critical temperature (Tc), as shown in the Right-sided graph. In contrast, in the case of the other normal materials, electrical resistance decreases as the temperature is lowered but does not disappear completely. | ||
The roadmap technology is the superconducting cable, which is the cable incorporated by some material that can show superconducting phenomenon (i.e., superconductors or superconducting material) to be designed for conducting electricity with zero resistance. (It is classified under the "Transporting Process (2)" and the "Energy (E)" categories in 5x5 matrix.) The technology is pivotal for mitigating energy losses inherent to electrical resistance, which is crucial for efficiency and operational cost reduction, especially in the context of long-distance power transmission. | The roadmap technology is the superconducting cable, which is the cable incorporated by some material that can show superconducting phenomenon (i.e., superconductors or superconducting material) to be designed for conducting electricity with zero resistance. (It is classified under the "Transporting Process (2)" and the "Energy (E)" categories in 5x5 matrix.) The technology is pivotal for mitigating energy losses inherent to electrical resistance, which is crucial for efficiency and operational cost reduction, especially in the context of long-distance power transmission. | ||
Line 14: | Line 16: | ||
[[File: 3SC_Section1_3.png| | [[File: 3SC_Section1_3.png|640px]] | ||
==Design Structure Matrix (DSM) Allocation== | ==Design Structure Matrix (DSM) Allocation== | ||
Line 31: | Line 33: | ||
We provide an Object-Process-Diagram (OPD) of the 3SC roadmap in the figure below. This diagram captures the main object of the roadmap (Superconducting Cable), including subsystem decompositions such as superconducting material wire and cooling system, and characterizes the Figures of Merits (FOMs) as well as the main processes (transporting) and state changes (electrical current from location A to location B without loss). Note that for room-temperature superconducting cables, the area enclosed by the red dashed-line square can be deleted from the OPD because it does not require the cooling medium. | We provide an Object-Process-Diagram (OPD) of the 3SC roadmap in the figure below. This diagram captures the main object of the roadmap (Superconducting Cable), including subsystem decompositions such as superconducting material wire and cooling system, and characterizes the Figures of Merits (FOMs) as well as the main processes (transporting) and state changes (electrical current from location A to location B without loss). Note that for room-temperature superconducting cables, the area enclosed by the red dashed-line square can be deleted from the OPD because it does not require the cooling medium. | ||
[[File: | [[File: 3SC_Section3_1_rev.png|600px|]] | ||
An Object-Process Language (OPL) of the roadmap scope, which corresponds with the above OPD, is shown as follows: | An Object-Process Language (OPL) of the roadmap scope, which corresponds with the above OPD, is shown as follows: | ||
Line 41: | Line 43: | ||
The table below shows a list of FOMs by which superconducting cable can be assessed. The FOMs are divided into two groups, compatible with the OPD. Group A shows the FOMs for the superconducting material layer, and Group B shows the FOMs for the superconducting cable, the technology itself we have chosen. To quantify how well a technology performs and how valuable the technology is for the users, it is indispensable to focus on the superconducting material layer, which is critical at the lower level of the system, as well as the cable. | The table below shows a list of FOMs by which superconducting cable can be assessed. The FOMs are divided into two groups, compatible with the OPD. Group A shows the FOMs for the superconducting material layer, and Group B shows the FOMs for the superconducting cable, the technology itself we have chosen. To quantify how well a technology performs and how valuable the technology is for the users, it is indispensable to focus on the superconducting material layer, which is critical at the lower level of the system, as well as the cable. | ||
[[File: | [[File: 3SC_Section4_1_rev.png|900px|]] | ||
Line 50: | Line 52: | ||
The critical temperature (Tc), the most prominent FOM, has evolved by following some stepping and rising trends. The journey of superconductors began with their discovery in 1911 by Heike Kamerlingh Onnes. Since then, the quest to elevate the critical temperature (Tc) has been relentless. Initially, the focus was on iron-based superconductors, represented by green dots in the following figure (It represents the historical trends of the critical temperature). | The critical temperature (Tc), the most prominent FOM, has evolved by following some stepping and rising trends. The journey of superconductors began with their discovery in 1911 by Heike Kamerlingh Onnes. Since then, the quest to elevate the critical temperature (Tc) has been relentless. Initially, the focus was on iron-based superconductors, represented by green dots in the following figure (It represents the historical trends of the critical temperature). | ||
[[File: 3SC_Section4_2_new.png| | [[File: 3SC_Section4_2_new.png|700px|]] | ||
<small>https://www.chemistryworld.com/news/room-temperature-superconductivity-finally-claimed-by-mystery-material/4012591.article</small> | <small>(https://www.chemistryworld.com/news/room-temperature-superconductivity-finally-claimed-by-mystery-material/4012591.article)</small> | ||
Line 63: | Line 65: | ||
'''Energy Efficiency Rate'''<br />[[File: 3SC_Section4_4.png|400px|thumb|middle|Energy Efficiency Rate Equation]] | '''Energy Efficiency Rate'''<br />[[File: 3SC_Section4_4.png|400px|thumb|middle|Energy Efficiency Rate Equation]] | ||
The Energy Efficiency Rate follows the governing equation shown in Right, which means a higher rate indicates minimal energy losses during transmission. For superconducting cables, the efficiency should ideally be close to 100%, indicating nearly zero energy loss during transmission. While over the years, there has been a consistent effort to improve their performance by following the trends of decreasing loss, especially against challenges such as short circuit faults, the present efficiency is around 95% at best. The rate has improved by 20% for 70 years, which means that the increase in the energy efficiency rate is about 0.3% per year(<small>Calculated based on the source: https://www.tepco.co.jp/corporateinfo/illustrated/electricity-supply/transmission-distribution-loss-j.html</small>). | The Energy Efficiency Rate follows the governing equation shown in Right, which means a higher rate indicates minimal energy losses during transmission. For superconducting cables, the efficiency should ideally be close to 100%, indicating nearly zero energy loss during transmission. While over the years, there has been a consistent effort to improve their performance by following the trends of decreasing loss, especially against challenges such as short circuit faults, the present efficiency is around 95% at best. The rate has improved by 20% for 70 years, which means that the increase in the energy efficiency rate is about 0.3% per year (<small>Calculated based on the source: https://www.tepco.co.jp/corporateinfo/illustrated/electricity-supply/transmission-distribution-loss-j.html (in Japanese)</small>). | ||
==Alignment with Company Strategic Drivers== | ==Alignment with Company Strategic Drivers== | ||
Line 95: | Line 97: | ||
The following table summarizes the significant parameters to explain the technology development in each project. Based on the coolant, consisting of the most costly subsystem in the technology, all projects adopt the high-temperature superconducting material (HTS) like BSCCO (Bismuth Strontium Calcium Copper Oxide) and YBCO (Yttrium Barium Copper Oxide) with liquid nitrogen as a cooling medium. The cable types, AC (alternating current) or DC (direct current) are different depending on each project goal and utility scenario, as described above. In general, each project has its target about the longer length of the cable, which can transmit electricity with high operating voltage and ampere with specific stability, scoping their future applications. The longer the cable is, the more complex the cable’s stability is. | The following table summarizes the significant parameters to explain the technology development in each project. Based on the coolant, consisting of the most costly subsystem in the technology, all projects adopt the high-temperature superconducting material (HTS) like BSCCO (Bismuth Strontium Calcium Copper Oxide) and YBCO (Yttrium Barium Copper Oxide) with liquid nitrogen as a cooling medium. The cable types, AC (alternating current) or DC (direct current) are different depending on each project goal and utility scenario, as described above. In general, each project has its target about the longer length of the cable, which can transmit electricity with high operating voltage and ampere with specific stability, scoping their future applications. The longer the cable is, the more complex the cable’s stability is. Using the information in the table, the tradespace (FOM chart (FOM1: Thermal Stability vs FOM2: Energy Capacity Density)) in the Technical Model Section shows the maps of the company and competitions. | ||
Line 111: | Line 113: | ||
* S. Yamaguchi et al., “Construction and 1st Experiment of the 500-meter and 1000-meter DC Superconducting Power Cable in Ishikari,” in Physics Procedia, Elsevier B.V., 2016, pp. 182–186. doi: 10.1016/j.phpro.2016.04.046. (https://www.sciencedirect.com/science/article/pii/S1875389216300463) | * S. Yamaguchi et al., “Construction and 1st Experiment of the 500-meter and 1000-meter DC Superconducting Power Cable in Ishikari,” in Physics Procedia, Elsevier B.V., 2016, pp. 182–186. doi: 10.1016/j.phpro.2016.04.046. (https://www.sciencedirect.com/science/article/pii/S1875389216300463) | ||
* https://ieeexplore.ieee.org/document/9366972 | * https://ieeexplore.ieee.org/document/9366972 | ||
* https://www.city.ishikari.hokkaido.jp/soshiki/kankyo/20401.html | * https://www.city.ishikari.hokkaido.jp/soshiki/kankyo/20401.html (in Japanese) | ||
3: Saint Petersburg | 3: Saint Petersburg | ||
Line 194: | Line 196: | ||
==Financial Model== | ==Financial Model== | ||
< | In order to construct the financial model, we assumed an electric power company that has the corporate strength to invest in the development of superconducting cables in the future, can install them by themselves and get benefit from the lower cost OPEX of superconducting cables. The model for the financial indicators is a major electric power company in Japan. | ||
<small>'''[Assumptions of the model company (electric power company)]''' | |||
* The total length of existing power transmission lines is 40,000km including 12,000km underground transmission lines. | |||
* Annual revenue is $40B and annual profit is 3%. | |||
* In other words, the annual revenue per 1km of transmission line is $1M/km. | |||
* As of 2023, the company is still operating an electric power business that utilizes existing power transmission lines. | |||
* The model company aim to develop a superconducting cable that can transport enough electrical current without any loss, securing sure profitability in the electricity market by 2040. The company aims to gradually increase profits by replacing their existing power transmission cables with new superconducting power transmission cables at a pace of 1,000 km in 3 years after 2040. They will make profits with the lower-cost OPEX of superconducting power transmission cables. As of 2060, there will be 33,000 km of conventional power transmission cables and 7,000 km of superconducting cables. | |||
'''[Premise of development contents]''' | |||
* We decided to promote development ourselves in line with the Strategic Driver described in Alignment with Company Strategic Drivers. However, we will proceed with excluding #4 the disruptive innovation room-temperature superconducting material layer that can be operated at atmospheric pressure. | |||
* The first phase is to develop high-temperature superconducting materials that are mechanically strong enough to be made into long cables by 2025 [R&D①]. The second phase is to design and test a cost-effective cryogenic cooling system to maintain cables at the temperatures for the superconducting phenomenon by 2030 [R&D②]. The third phase is to engineer and establish the technologies for some cable joints and quenching subsystems to make our roadmap technology much practicable in the market by 2040 [R&D③]. | |||
* In achieving R&D ①, we will focus on developing cables of sufficient length with an eye to extending the distance with the premise of future cable-to-cable connections. As mentioned in the section on Positioning of Company vs. Competition, multiple prototype tests have already been conducted that will lead to the practical use of cables of a certain length, so this is a wrap-up development to ensure future prospects, it is assumed that a budget of $666M/year will be invested for two years starting in 2024. | |||
* Next, R&D ② is the most critical point for future development continuation, as the current issue with superconducting cables is the difficulty of ensuring profitability, including the cooling system. Therefore, this phase needs to be the most budget-invested phase. It is assumed that a budget of $2666M/year will be invested for 5 years starting in 2026. | |||
* Final R&D ③ is an important development not only from the viewpoint of system feasibility but also from the viewpoint of robustness. It is assumed that a budget of $1333M/year will be invested for nine years starting in 2031. | |||
'''[Other assumptions for the model construction]''' | |||
* The unit price for underground power transmission lines (long distance) is $6.66K/km. [1] | |||
* In the future, the unit price of superconducting cables is expected to improve in performance and decrease in price to approximately $20/kAm@77K. The price of $20/kAm is almost the same as copper wire. [2] Therefore, the unit price of the superconducting cable itself is set at $6.66K/km, the same as the underground cable (long distance). | |||
* The introduction cost of LN2 cooling system is set at $153.33K/km. We calculated the power transmission specifications by assuming 77kV and 10kA [3] and multiplying them by "2.3" [4], which is the difference in the price index from when the paper was written. | |||
* The standard price of a 77kV transformer is approximately $666.66K/equipment [5]. Assuming that one unit is installed every 10km, the cost will be $66.66K/km. | |||
* CAPEX generated after 2040 is capital expenditure associated with cable replacement. Since this is a replacement work, it is assumed that most of the existing power transmission equipment (power plants, etc.) can be used as is, but the superconducting cable itself, the cooling system itself, and the addition/replacement of a transformer with a view to handling higher voltages will be considered as CAPEX. | |||
* If conventional power transmission cables are replaced with superconducting cables (DC), the transmission loss cost will be ▲$420K/km. [6] This reduction in cost contributes to lower OPEX costs after replacing with superconducting cables. | |||
* Conduct financial analysis up to 2060. Revenue, OPEX, and Profit (throughout the entire period) from the power business using existing transmission lines, expenditures related to the development of superconducting cables (until 2040), CAPEX related to the introduction of superconducting cables (from 2040), and Revenue, OPEX, and Profit (from 2040) that can be earned from the power business through superconducting cable transmission are included. | |||
* Discount rate is set to 5%.</small> | |||
[[File:3SC Section8 1 rev1.png|750px|]] | |||
[[File:3SC Section8 2 rev2.png|750px|]] | |||
'''[Result of analysis]''' | |||
This financial model is based on the step-by-step and planned development and implementation of superconducting cables. This analysis shows that the Cumulative DCF will turn positive in the 9th year (2048 C.E.) after entering the superconducting cable implementation phase. After that, until 2060, Cumulative DCF will further increase as the replacement rate to superconducting cables progresses. The final Net Present Value is estimated to be $7,916M, indicating a sufficient return on investment. Since the initial R&D investment is large, the development of superconducting cables may be worthwhile investment for companies that already have a sufficient business base, such as the company used as the model for this analysis, or if there is an environment where government subsidies can be effectively utilized. | |||
<small> '''[Reference]''' | |||
* [1] Electricity and Gas Market Surveillance Commission, “Analysis of procurement unit price and construction work carried out using construction cost contributions”, 2019 (in Japanese) (https://www.emsc.meti.go.jp/info/public/pdf/20190328001h.pdf) | |||
* [2] [6] M. Hirose et al., “Consideration of commercialization of high-temperature superconductivity”, SEI Technical Review (2006) (in Japanese) (https://sei.co.jp/technology/tr/pdf/sei10449.pdf) | |||
* [3] Seijiro Ihara and Takashi Horigome, “Consideration on the Economy for Superconducting DC Power Transmission”, Cryogenics Engineering (1970) (in Japanese) (https://www.jstage.jst.go.jp/article/jcsj1966/5/1/5_1_8/_pdf/-char/ja) | |||
* [4] Bank of Japan Research and Statistics Department, “Corporate Price Index” | |||
* [5] Organization for Cross-regional Coordination of Transmission Operators, Japan, “Publication of standard unit prices for power transmission and substation equipment”, 2016 (in Japanese) (https://www.occto.or.jp/access/oshirase/2015/files/20160329_tanka_kouhyou.pdf) | |||
==List of R&D Projects and Prototypes== | ==List of R&D Projects and Prototypes== | ||
Line 217: | Line 261: | ||
* S. Yamaguchi et al., “Construction and 1st Experiment of the 500-meter and 1000-meter DC Superconducting Power Cable in Ishikari,” in Physics Procedia, Elsevier B.V., 2016, pp. 182–186. doi: 10.1016/j.phpro.2016.04.046. (https://www.sciencedirect.com/science/article/pii/S1875389216300463) | * S. Yamaguchi et al., “Construction and 1st Experiment of the 500-meter and 1000-meter DC Superconducting Power Cable in Ishikari,” in Physics Procedia, Elsevier B.V., 2016, pp. 182–186. doi: 10.1016/j.phpro.2016.04.046. (https://www.sciencedirect.com/science/article/pii/S1875389216300463) | ||
* https://ieeexplore.ieee.org/document/9366972 | * https://ieeexplore.ieee.org/document/9366972 | ||
* https://www.city.ishikari.hokkaido.jp/soshiki/kankyo/20401.html | * https://www.city.ishikari.hokkaido.jp/soshiki/kankyo/20401.html (in Japanese) | ||
3: Saint Petersburg | 3: Saint Petersburg |
Latest revision as of 16:07, 21 December 2023
Time Stamp: 5 December 2023
Technology Roadmap Sections and Deliverables
- 3SC - Superconducting Cable
Roadmap Overview
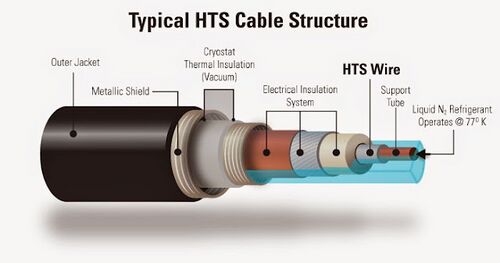
The feature of the superconductivity phenomenon, the basis of this roadmap technology (3SC - Superconducting Cable), is an unusual property of certain substances, including metals, alloys, and ceramics, in which electrical resistance drops to zero when the temperature is reduced below a critical temperature (Tc), as shown in the Right-sided graph. In contrast, in the case of the other normal materials, electrical resistance decreases as the temperature is lowered but does not disappear completely.
The roadmap technology is the superconducting cable, which is the cable incorporated by some material that can show superconducting phenomenon (i.e., superconductors or superconducting material) to be designed for conducting electricity with zero resistance. (It is classified under the "Transporting Process (2)" and the "Energy (E)" categories in 5x5 matrix.) The technology is pivotal for mitigating energy losses inherent to electrical resistance, which is crucial for efficiency and operational cost reduction, especially in the context of long-distance power transmission.
In general, there are three types of superconductors: low temperature superconductors, high temperature superconductors and room temperature superconductors. The Right-sided figure shows the typical depiction of high temperature superconducting cable, incorporating high temperature superconducting materials, which is designed to conduct electricity with zero resistance by using liquid nitrogen to cool the superconductors up to certain enough temperature(e.g., to 77k) and activate the superconductivity phenomenon. Compared with the traditional low temperature superconducting materials, which necessitate even lower temperatures, typically requiring liquid helium, high temperature superconducting cable can perform the superconductive state with relatively higher temperatures inside the cable. It means that the high temperature superconducting cable is advantageous regarding the cooling costs and the availability of coolants.
The system concept of the high temperature superconducting cable (including a cooling system) transporting electrical current without loss is as follows. Typically, High Temperature Superconducting (HTS) wires, consisting of superconducting materials, are placed in a pipe (cryostat thermal insulation) with a vacuum, which thermally isolates the superconductor from the remaining environment. Inside the pipe is the liquid nitrogen flow path. The technology requires special cable joints and specific cable termination for extreme temperature differences and a permanent cooling system for keeping the cryostat. Note: If room temperature superconductors that operate at atmospheric pressure are discovered in the future, the need for a cooling system can be eliminated. That is one of the ultimate technologies; however, they are still in the basic research stage.
Design Structure Matrix (DSM) Allocation
The 3SC-tree that we can extract from the DSM above shows us that the Superconducting Cable (3SC) is part of a larger system initiative on Superconducting Power Transmission (2SPT), and Superconducting Power Transmission is also part of a larger initiative on achieving Efficient Electric Power Supply (1EEPS).
As subsystem level of the Superconducting Power Transmission, other than Superconducting Cable, the following are required: Power Output Control (3POC), Power Generation (3PG), Power Substation (3PS) and Power Conversion (3PC).
Superconducting Cable requires the following key enabling technologies at the component level: Superconducting Wires (4SW), Cooling (4COL), Thermal Insulation (4TI), Electrical Insultation (4EI) and Magnet Field Insulation (4MFI). These five are the most common technology components to apply the phenomenon of superconductivity to the power transmission cable.
Roadmap Model using OPM
We provide an Object-Process-Diagram (OPD) of the 3SC roadmap in the figure below. This diagram captures the main object of the roadmap (Superconducting Cable), including subsystem decompositions such as superconducting material wire and cooling system, and characterizes the Figures of Merits (FOMs) as well as the main processes (transporting) and state changes (electrical current from location A to location B without loss). Note that for room-temperature superconducting cables, the area enclosed by the red dashed-line square can be deleted from the OPD because it does not require the cooling medium.
An Object-Process Language (OPL) of the roadmap scope, which corresponds with the above OPD, is shown as follows:
Figures of Merit
The table below shows a list of FOMs by which superconducting cable can be assessed. The FOMs are divided into two groups, compatible with the OPD. Group A shows the FOMs for the superconducting material layer, and Group B shows the FOMs for the superconducting cable, the technology itself we have chosen. To quantify how well a technology performs and how valuable the technology is for the users, it is indispensable to focus on the superconducting material layer, which is critical at the lower level of the system, as well as the cable.
The important FOM trends over time dFOM/dt as well as some of the key governing equations that underpin the technology are summarized as follows:
Critical Temperature and operating pressure
The critical temperature (Tc), the most prominent FOM, has evolved by following some stepping and rising trends. The journey of superconductors began with their discovery in 1911 by Heike Kamerlingh Onnes. Since then, the quest to elevate the critical temperature (Tc) has been relentless. Initially, the focus was on iron-based superconductors, represented by green dots in the following figure (It represents the historical trends of the critical temperature).
The then governing equation in superconductivity was assumed by BCS theory, a fundamental one based on the following equation:
A monumental shift occurred in 1986 with the emergence of high-temperature superconductors like LaBaCuO, symbolized by blue diamonds, which was beyond the BCS theory and its governing equation. While Nb-based superconductors had a Tc of 20-30K, the high-temperature variant YBCO soared beyond 100K, surpassing even the liquid nitrogen temperature (77K). This achievement held immense practical implications. The subsequent years saw the discovery of superconductors nearing room temperature, though their real-world application is constrained by the need for extremely high pressures, often exceeding 100GPa (i.e., The operating pressure is another FOM of the technology). At present, the critical temperature is found through simulations and experiments, not yet elucidated the governing equations. To summarize the trends, the critical temperature has risen by 280 Kelvin for 110 years since 1911, which means that the rate of critical temperature increase is 2.5 Kelvin per year.
Energy Efficiency Rate
The Energy Efficiency Rate follows the governing equation shown in Right, which means a higher rate indicates minimal energy losses during transmission. For superconducting cables, the efficiency should ideally be close to 100%, indicating nearly zero energy loss during transmission. While over the years, there has been a consistent effort to improve their performance by following the trends of decreasing loss, especially against challenges such as short circuit faults, the present efficiency is around 95% at best. The rate has improved by 20% for 70 years, which means that the increase in the energy efficiency rate is about 0.3% per year (Calculated based on the source: https://www.tepco.co.jp/corporateinfo/illustrated/electricity-supply/transmission-distribution-loss-j.html (in Japanese)).
Alignment with Company Strategic Drivers
The following table shows our strategic drivers and the alignment of those drivers with this roadmap. As the table shows, the roadmap for superconducting cable technology is insufficient by itself to enable other superconducting application technologies, which lead the research and development of superconducting material itself. That is, other applications could develop our roadmap, adding different perspectives about the strategic drivers for the technology.
# | Strategic Driver | Alignment and Targets |
---|---|---|
1 | To develop a long enough superconducting material layer with mechanical strength to maintain its superconducting properties at atmospheric pressure when subjected to bending, twisting, or other physical forces by 2025. | The 3SC technology roadmap will target to make the superconducting material layer at least 1 mile (1.6km), which can transmit more than 10kA electricity at 650kV by DC power cables. In theory, the transmission distance can be extended more with future cable connections.
This strategic driver is aligned but not fully covered with the technology roadmap for the superconducting ”cable”, because it depends on the kinds of superconducting material applications. |
2 | To develop an affordable cooling system for insulating the heat of superconducting cables that will launch a profitable marketplace where we have a distinct advantage in the superconducting cable market instead of the regular electrical cable market by 2030. | The 3SC technology roadmap will develop and test the cooling system, leading to the reduction of the total operating cost compared to the regular electrical cables by 40% in total system.
This strategic driver is aligned with the technology roadmap as this target corresponds with FOM #B5. |
3 | To develop a superconducting cable with joints and quenching subsystem that can be safely connected with other cables, electric power substations and factories, where the technology will replace existing regular electrical cables by 2040. | The 3SC technology roadmap will help to develop the applicable quenching subsystem by detecting the predictors of quenching enough before the system’s failures and stopping the electricity flows.
This strategic driver is partially aligned with the 3SC roadmap, though different breakthroughs about quenching subsystems could impact the roadmap. |
4 | To develop a room-temperature superconducting material layer that can be operated at atmospheric pressure to remove the cooling system, where all existing copper cables could be replaced by 2100. | The 3SC technology roadmap could scope the discovery of the room-temperature superconducting material layer, but such materials (that are regarded as disruptive innovation) have not been discovered at all as of 2023.
This strategic driver is aligned with the 3SC roadmap as this target corresponds with FOM #A1~A7. |
Positioning of Company vs. Competition
In the competitive landscape, the “company” faces rivalry from several key players: Ampa City (Germany), Ishikari (Japan), Saint Petersburg (Russia), HYDRA (US), and Shingal (South Korea). Given that some projects are financed or managed by government or national institutes*, the superconducting cable is regarded as a technology that includes some high-level technical challenges and/or implies difficulties in profitability. Also, the technology, highly related to the electricity system flexibility, is expected to contribute to developing high-capacity transmission networks to meet the national/regional big goals about the energy balance. Therefore, for overviewing the competitive environment, it is necessary not only to compare the technological properties but also to understand each project’s background.
(*Ampa City: Funded by the German Government, Ministry of Economics and Technology, Ishikari: Sponsored by the National Institute of Advanced Industrial Science and Technology, Japan, HYDRA: Operated by Oak Ridge National Laboratory, the Department of Energy, US)
The following table summarizes the significant parameters to explain the technology development in each project. Based on the coolant, consisting of the most costly subsystem in the technology, all projects adopt the high-temperature superconducting material (HTS) like BSCCO (Bismuth Strontium Calcium Copper Oxide) and YBCO (Yttrium Barium Copper Oxide) with liquid nitrogen as a cooling medium. The cable types, AC (alternating current) or DC (direct current) are different depending on each project goal and utility scenario, as described above. In general, each project has its target about the longer length of the cable, which can transmit electricity with high operating voltage and ampere with specific stability, scoping their future applications. The longer the cable is, the more complex the cable’s stability is. Using the information in the table, the tradespace (FOM chart (FOM1: Thermal Stability vs FOM2: Energy Capacity Density)) in the Technical Model Section shows the maps of the company and competitions.
Reference
1: Ampa City
- M. Stemmle, F. Merschel, M. Noe, and A. Hobl, “Ampacity project - worldwide first superconducting cable and fault current limiter installation in a German city center,” in 22nd International Conference and Exhibition on Electricity Distribution (CIRED 2013), Institution of Engineering and Technology, 2013, pp. 0742–0742. doi: 10.1049/cp.2013.0905. (http://cired.net/publications/cired2015/papers/CIRED2015_0678_final.pdf)
- https://www.tdworld.com/overhead-distribution/article/20964180/the-ampacity-project
2: Ishikari
- S. Yamaguchi et al., “Construction and 1st Experiment of the 500-meter and 1000-meter DC Superconducting Power Cable in Ishikari,” in Physics Procedia, Elsevier B.V., 2016, pp. 182–186. doi: 10.1016/j.phpro.2016.04.046. (https://www.sciencedirect.com/science/article/pii/S1875389216300463)
- https://ieeexplore.ieee.org/document/9366972
- https://www.city.ishikari.hokkaido.jp/soshiki/kankyo/20401.html (in Japanese)
3: Saint Petersburg
- V. Sytnikov, A. Kashcheev, M. Dubinin, V. Karpov, and T. Ryabin, “Test Results of the Full-Scale HTS Transmission Cable Line (2.4 Km) for the St. Petersburg Project,” IEEE Transactions on Applied Superconductivity, vol. 31, no. 5, Aug. 2021, doi: 10.1109/TASC.2021.3063067. (https://ieeexplore.ieee.org/stamp/stamp.jsp?tp=&arnumber=9366972)
- https://globalenergyprize.org/en/2020/04/29/saint-petersburg-will-receive-electricity-trough-the-worlds-longest-superconductor-line/#:~:text=The%20HTS%20CL%20is%20based,certain%20temperatures%2065%2D80%20K.
4: HYDRA
5: Shingal
- C. Lee et al., “Progress of the first commercial project of higherature superconducting cables by KEPCO in Korea,” Supercond Sci Technol, vol. 33, no. 4, 2020, doi: 10.1088/1361-6668/ab6ec3. (https://iopscience.iop.org/article/10.1088/1361-6668/ab6ec3/meta#:~:text=Korea%20Electric%20Power%20Corporation%20(KEPCO,operations%20are%20ready%20to%20start)
Technical Model
The following table shows the main technological decision variables on Superconducting Cable that would affect Thermal Stability and Energy Capacity Density.
Thermal Stability (St) is defined here as the ratio of the difference between the critical temperature (Tc) and the refrigerant temperature to the refrigerant temperature rise in one cooling system cycle. The higher the value of St, the higher the thermal stability. If St is less than 1, the superconducting state cannot be maintained in theory and the system will not function. The unit of St is a comparison between K and is dimensionless. The formula assumes an "equilibrium state" in which the superconducting material has been sufficiently cooled by the refrigerant and is already at the same temperature as the refrigerant. Below shows the fomula of Thermal Stability (St) and its analytical partial derivatives with respect to variables.
Energy Capacity Density (De) represents the amount of energy that can be transmitted per second per cross section of a superconducting cable, and the unit is [MVA/m^2]. A mathematical formula was constructed assuming DC power transmission, which makes it easy to utilize the characteristics of large-capacity power transmission using high-temperature superconducting cables. Also, for this FOM, as in the case of St, the analytical partial derivatives with respect to variables is calculated as follows.
As indicated in the Positioning of Company vs. Competition section, information on five case studies as model projects from among the projects that have been implemented to date to commercialize high-temperature superconducting cables has been collected. Among the past projects mentioned above, the project #5 (Shingal) is designated as a representative design and conduct sensitivity analysis. The following table is the design characteristics of the Shingal Project.
Many values were determined from the project data, while the asterisks in the table above mean assumed values. Given a particular design vector xo (Shingal Project) with values shown in the table above, the normalized sensitivity analysis can be conducted. This is to calculate the percentage increase in St and De when each parameter increases by 1% in the representative model.
The results of the normalized sensitivity analysis for Thermal Stability (St) are shown in the Tornado Diagram below.
Based on the tornado diagram above, it is shown that the most sensitive parameter for Thermal Stability (St) is Tc. Following that, Tr, φ, and To have the greatest influence for St. It can be seen from this graph that in order to maintain a stable superconducting state, it is effective to increase critical Temperature (Tc) by keeping the difference from the refrigerant temperature as large as possible. Tc is determined as a value unique to superconducting materials, so it can be said that cable systems using superconducting materials with higher Tc are advantageous from the perspective of thermal stability (St).
On the other hand, the analysis results for Energy Capacity Density (De) are shown in another Tornado Diagram below. From φ, I, and V, the parameter with the highest sensitivity is the cable diameter φ in this model.
Having decided to use some of the figures obtained from the past five projects mentioned above to ensure the validity of the figures as much as possible for a tradespase. Rated voltage [V], rated current [I] and cable length [L] can be determined directly from each past project. Additionally, the critical temperature of superconducting material [Tc], which is an eigenvalue, can be determined from information on the high-temperature superconducting material used in each project. Furthermore, since liquid nitrogen is widely used as a refrigerant for high-temperature superconducting materials, its characteristic values such as refrigerant temperature [Tr], heat capacity of refrigerant [c], and density of refrigerant [r] can also be determined.
In light of these circumstances, this time in our tradespace, we would obtain parameters that can be cited and identified from five past projects as described above, and then set fixed or multiple values for each. Of the remaining parameters, based on the results of normalized sensitivity analysis, multiple choises (four or three respectively) are given for cable diameter [φ] and circulation speed of refrigerant in the cable [v], which have high sensitivity and many design options. For outside temperature [To], thermal insulation thickness [d], and thermal conductivity of insulation material [k], which do not apply to all the above, fixed values were adopted in this case.
The list of design decisions identified according to the above policy is as follows. There is total 60 combinations of design decisions, which also shows the morphological matrix. The DD5-7 is same with the representative design we used for the normalized sensitivity analysis. The asterisks in the figure below means assumed values.
The tradespace can be obtained by substituting the combination of these parameters into the calculation formulas for Tc and De, and plotting it. It is plotted with FOM1 (St: Thermal Stability) on the vertical axis and FOM2 (De: Energy Capacity Density) on the horizontal axis.
By exploring the tradespace above we can get insights as following:
- The higher both De and St, the more desirable the index. Therefore, the utopia point is placed at the top right of the trade space graph.
- Regarding the relationship between De and St, in all five projects, St tended to decrease as De increased. For example, the green trend line of the PJ #4 visually represents this relationship and tendency.
- It was found that as the cable diameter Φ increases, St increases, but De decreases. The reason why St increases as Φ increases is as follows; The increase in volume that prevents the temperature rise of the refrigerant due to heat exchange becomes dominant to the increase in cable surface area, which is the driver of heat exchange between the refrigerant and the outside.
- FOM1 (St) increases as the circulation speed of refrigerant v increases. When v is low, FOM1 (St) also become low even for the same value of FOM2 (De).
- In some design choices in projects other than the PJ #4, there were combinations that were below the system lower limit of 1 for Ts in terms of maintaining superconductivity. These are design combinations that do not actually work.
Financial Model
In order to construct the financial model, we assumed an electric power company that has the corporate strength to invest in the development of superconducting cables in the future, can install them by themselves and get benefit from the lower cost OPEX of superconducting cables. The model for the financial indicators is a major electric power company in Japan.
[Assumptions of the model company (electric power company)]
- The total length of existing power transmission lines is 40,000km including 12,000km underground transmission lines.
- Annual revenue is $40B and annual profit is 3%.
- In other words, the annual revenue per 1km of transmission line is $1M/km.
- As of 2023, the company is still operating an electric power business that utilizes existing power transmission lines.
- The model company aim to develop a superconducting cable that can transport enough electrical current without any loss, securing sure profitability in the electricity market by 2040. The company aims to gradually increase profits by replacing their existing power transmission cables with new superconducting power transmission cables at a pace of 1,000 km in 3 years after 2040. They will make profits with the lower-cost OPEX of superconducting power transmission cables. As of 2060, there will be 33,000 km of conventional power transmission cables and 7,000 km of superconducting cables.
[Premise of development contents]
- We decided to promote development ourselves in line with the Strategic Driver described in Alignment with Company Strategic Drivers. However, we will proceed with excluding #4 the disruptive innovation room-temperature superconducting material layer that can be operated at atmospheric pressure.
- The first phase is to develop high-temperature superconducting materials that are mechanically strong enough to be made into long cables by 2025 [R&D①]. The second phase is to design and test a cost-effective cryogenic cooling system to maintain cables at the temperatures for the superconducting phenomenon by 2030 [R&D②]. The third phase is to engineer and establish the technologies for some cable joints and quenching subsystems to make our roadmap technology much practicable in the market by 2040 [R&D③].
- In achieving R&D ①, we will focus on developing cables of sufficient length with an eye to extending the distance with the premise of future cable-to-cable connections. As mentioned in the section on Positioning of Company vs. Competition, multiple prototype tests have already been conducted that will lead to the practical use of cables of a certain length, so this is a wrap-up development to ensure future prospects, it is assumed that a budget of $666M/year will be invested for two years starting in 2024.
- Next, R&D ② is the most critical point for future development continuation, as the current issue with superconducting cables is the difficulty of ensuring profitability, including the cooling system. Therefore, this phase needs to be the most budget-invested phase. It is assumed that a budget of $2666M/year will be invested for 5 years starting in 2026.
- Final R&D ③ is an important development not only from the viewpoint of system feasibility but also from the viewpoint of robustness. It is assumed that a budget of $1333M/year will be invested for nine years starting in 2031.
[Other assumptions for the model construction]
- The unit price for underground power transmission lines (long distance) is $6.66K/km. [1]
- In the future, the unit price of superconducting cables is expected to improve in performance and decrease in price to approximately $20/kAm@77K. The price of $20/kAm is almost the same as copper wire. [2] Therefore, the unit price of the superconducting cable itself is set at $6.66K/km, the same as the underground cable (long distance).
- The introduction cost of LN2 cooling system is set at $153.33K/km. We calculated the power transmission specifications by assuming 77kV and 10kA [3] and multiplying them by "2.3" [4], which is the difference in the price index from when the paper was written.
- The standard price of a 77kV transformer is approximately $666.66K/equipment [5]. Assuming that one unit is installed every 10km, the cost will be $66.66K/km.
- CAPEX generated after 2040 is capital expenditure associated with cable replacement. Since this is a replacement work, it is assumed that most of the existing power transmission equipment (power plants, etc.) can be used as is, but the superconducting cable itself, the cooling system itself, and the addition/replacement of a transformer with a view to handling higher voltages will be considered as CAPEX.
- If conventional power transmission cables are replaced with superconducting cables (DC), the transmission loss cost will be ▲$420K/km. [6] This reduction in cost contributes to lower OPEX costs after replacing with superconducting cables.
- Conduct financial analysis up to 2060. Revenue, OPEX, and Profit (throughout the entire period) from the power business using existing transmission lines, expenditures related to the development of superconducting cables (until 2040), CAPEX related to the introduction of superconducting cables (from 2040), and Revenue, OPEX, and Profit (from 2040) that can be earned from the power business through superconducting cable transmission are included.
- Discount rate is set to 5%.
[Result of analysis]
This financial model is based on the step-by-step and planned development and implementation of superconducting cables. This analysis shows that the Cumulative DCF will turn positive in the 9th year (2048 C.E.) after entering the superconducting cable implementation phase. After that, until 2060, Cumulative DCF will further increase as the replacement rate to superconducting cables progresses. The final Net Present Value is estimated to be $7,916M, indicating a sufficient return on investment. Since the initial R&D investment is large, the development of superconducting cables may be worthwhile investment for companies that already have a sufficient business base, such as the company used as the model for this analysis, or if there is an environment where government subsidies can be effectively utilized.
[Reference]
- [1] Electricity and Gas Market Surveillance Commission, “Analysis of procurement unit price and construction work carried out using construction cost contributions”, 2019 (in Japanese) (https://www.emsc.meti.go.jp/info/public/pdf/20190328001h.pdf)
- [2] [6] M. Hirose et al., “Consideration of commercialization of high-temperature superconductivity”, SEI Technical Review (2006) (in Japanese) (https://sei.co.jp/technology/tr/pdf/sei10449.pdf)
- [3] Seijiro Ihara and Takashi Horigome, “Consideration on the Economy for Superconducting DC Power Transmission”, Cryogenics Engineering (1970) (in Japanese) (https://www.jstage.jst.go.jp/article/jcsj1966/5/1/5_1_8/_pdf/-char/ja)
- [4] Bank of Japan Research and Statistics Department, “Corporate Price Index”
- [5] Organization for Cross-regional Coordination of Transmission Operators, Japan, “Publication of standard unit prices for power transmission and substation equipment”, 2016 (in Japanese) (https://www.occto.or.jp/access/oshirase/2015/files/20160329_tanka_kouhyou.pdf)
List of R&D Projects and Prototypes
This list compiles R&T and prototype projects by technology area. Each project type is categorized into R&D, Breakthrough, Platform, or Derivative. Projects #1 through #5 are actual projects. While there are numerous demonstrations using superconductors, the production of superconducting cables and the need for cooling systems mean that many are on a relatively large scale. Therefore, the prototypes are generally produced using already established methods, and their scope is primarily Derivative. The priority was determined based on the impact on the Possibility of the technology and its Figure of Merit (FOM).
The following diagram organizes each project within the categories of R&D, Breakthrough, Platform, and Derivative, based on the list. Room Temperature Superconductors (A) can be used without cooling, but they have not yet been discovered. If discovered, their applications could extend beyond power cables to quantum computers, MRI machines, and energy storage devices, potentially leading to disruptive innovation. Although this technology is being explored by research institutions worldwide, its possibility is unpredictable (assumed low), making it impractical for us, as a power company, to invest heavily in it as R&D. Also, Room-Temperature Superconductors that require high pressure ((B1)) for operation have been discovered, but the required pressure is extremely high, and their practicality remains uncertain. Therefore, our Financial Model does not include a budget for Room-Temperature Superconductors.
Research into high-temperature superconductors (C) and superconducting structures usable in high magnetic fields (D), both of which hold promising applications, is actively underway. The current technology has already advanced to a level sufficient for application in superconducting cables, hence a significant investment in these areas is not planned. On the other hand, by investing in cooling systems (E) and cable interconnection technologies (F), which are nearing practical levels, we aim for early commercialization.
Reference
1: Ampa City
- M. Stemmle, F. Merschel, M. Noe, and A. Hobl, “Ampacity project - worldwide first superconducting cable and fault current limiter installation in a German city center,” in 22nd International Conference and Exhibition on Electricity Distribution (CIRED 2013), Institution of Engineering and Technology, 2013, pp. 0742–0742. doi: 10.1049/cp.2013.0905. (http://cired.net/publications/cired2015/papers/CIRED2015_0678_final.pdf)
- https://www.tdworld.com/overhead-distribution/article/20964180/the-ampacity-project
2: Ishikari
- S. Yamaguchi et al., “Construction and 1st Experiment of the 500-meter and 1000-meter DC Superconducting Power Cable in Ishikari,” in Physics Procedia, Elsevier B.V., 2016, pp. 182–186. doi: 10.1016/j.phpro.2016.04.046. (https://www.sciencedirect.com/science/article/pii/S1875389216300463)
- https://ieeexplore.ieee.org/document/9366972
- https://www.city.ishikari.hokkaido.jp/soshiki/kankyo/20401.html (in Japanese)
3: Saint Petersburg
- V. Sytnikov, A. Kashcheev, M. Dubinin, V. Karpov, and T. Ryabin, “Test Results of the Full-Scale HTS Transmission Cable Line (2.4 Km) for the St. Petersburg Project,” IEEE Transactions on Applied Superconductivity, vol. 31, no. 5, Aug. 2021, doi: 10.1109/TASC.2021.3063067. (https://ieeexplore.ieee.org/stamp/stamp.jsp?tp=&arnumber=9366972)
- https://globalenergyprize.org/en/2020/04/29/saint-petersburg-will-receive-electricity-trough-the-worlds-longest-superconductor-line/#:~:text=The%20HTS%20CL%20is%20based,certain%20temperatures%2065%2D80%20K.
4: HYDRA
5: Shingal
- C. Lee et al., “Progress of the first commercial project of higherature superconducting cables by KEPCO in Korea,” Supercond Sci Technol, vol. 33, no. 4, 2020, doi: 10.1088/1361-6668/ab6ec3. (https://iopscience.iop.org/article/10.1088/1361-6668/ab6ec3/meta#:~:text=Korea%20Electric%20Power%20Corporation%20(KEPCO,operations%20are%20ready%20to%20start)
Key Publications, Presentations and Patents
Our review of patents and academic papers aims to facilitate the pragmatic implementation of superconducting cables, by identifying the three technological domains as follows:
- 1. Enhancement of Critical Current Density in Superconducting Materials under High Magnetic Fields
- 2. Techniques for Lengthening and Cooling Superconducting Cables for Practical Use
- 3. Technology for Superconducting Cable Failure Modes
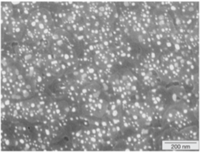
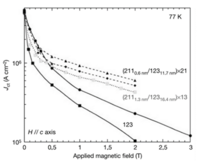
1. Enhancement of Critical Current Density in Superconducting Materials under High Magnetic Fields
- Key publication
Superconducting materials experience degradation under high magnetic fields due to the generation of self-magnetic fields and exposure to external magnetic fields. T. Haugan's research revealed that introducing impurities to superconductors can mitigate this degradation. In the YBCO superconducting material, as visualized in the SEM image (upper figure), bromine (Br) impurities ranging from nanometers to tens of nanometers are present. The authors modified the typical YBCO composition, resulting in enhanced critical current densities under magnetic fields (lower figure).
Source: T. Haugan, P. N. Barnes, R. Wheeler, F. Meisenkothen, and M. Sumption, “Addition of nanoparticle dispersions to enhance flux pinning of the YBa2Cu3O7-x superconductor,” Nature, vol. 430, no. 7002, pp. 867–870, Aug. 2004, doi: 10.1038/nature02792. (https://www.nature.com/articles/nature02792)
- Key patent
Sumitomo Electric Industries patented a method (PCT/JP2017/039310) to enhance critical current density by introducing bromine (Br) to YBCO superconducting materials.
Source: T. MOTOKI, J. SHIMOYAMA, G. HONDA, T. NAGAISHI, Sumitomo Electric Industries, Ltd., Osaka-shi, Osaka (JP), Aug. 12, 2019, “OXIDE SUPERCONDUCTING THIN FILM MATERIAL, OXIDE SUPERCONDUCTING THIN FILM WIRE, AND METHOD FOR MANUFACTURING OXIDE SUPERCONDUCTING THIN FILM”, PCT/JP2017/039310
2. Techniques for Lengthening and Cooling Superconducting Cables for Practical Use
- Key publication
For superconducting cables to be practical, addressing the challenge of lengthening is vital. Factory-shipped superconducting cables have inherent length limitations due to their diameter and manufacturing constraints. S. Mukoyama's experiment showcased the potential of a 50-meter high-temperature superconducting cable, emphasizing adjustments in impedance to minimize AC transmission losses.
Source: S. Mukoyama et al., “50-m long HTS conductor for power cable,” IEEE Transactions on Applied Superconductivity, vol. 7, no. 2, pp. 1069–1072, Jun. 1997, doi: 10.1109/77.614709. (https://ieeexplore.ieee.org/document/614709)
- Key patent
R. Fukuda's patent (H01B 12/16 (2013.01); H02G I/081(2013.01); H02G 15/34 (2013.01)) shows the invention of the cooling approach for superconducting cables. Instead of the traditional design where the core is encased within a coolant flow path, his design strategically places an external coolant channel separate from the core. This innovation not only allows for the creation of longer superconducting cores but also optimizes cooling efficiency. By ensuring uniform and effective temperature maintenance across the cable, especially for extended lengths, Fukuda's approach provides a balanced and pragmatic solution that addresses both design flexibility and operational stability in superconducting cables.
Source: R. FUKUDA, Feb. 21, 2014, SUPERCONDUCTING CABLE,SUPERCONDUCTING CABLE LINE,METHOD OF INSTALLING SUPERCONDUCTING CABLE, AND METHOD OF OPERATING SUPERCONDUCTING CABLE LINE, H01B 12/16 (2013.01); H02G I/081(2013.01); H02G 15/34 (2013.01)
3. Technology for Superconducting Cable Failure Modes
- Key publication
The "quench" phenomenon, a sudden transition from a superconducting state to a non-superconducting state, poses significant risks to the whole system's safety. Several factors, such as heat or material defects, can trigger quench. Yawei Wang's research using high-temperature superconducting REBCO thin films provides insights into predicting quench events and understanding their causes.
Source: Y. Wang, J. Zheng, Z. Zhu, M. Zhang, and W. Yuan, “Quench behavior of high-temperature superconductor (RE)Ba2Cu3Ox CORC cable,” J Phys D Appl Phys, vol. 52, no. 34, Jun. 2019, doi: 10.1088/1361-6463/ab1e2c. (https://iopscience.iop.org/article/10.1088/1361-6463/ab1e2c)
- Key patent
M. Takayasu's patent (G01K 13/006) proposes quench detection using strain measurements from multiple pressure sensors, offering a potential solution to this challenge, as shown in the right figure.
Source: M. TAKAYASU, Oct. 20 , 2020, “DISTRIBUTED-SENSOR QUENCH DETECTION METHOD FOR SUPERCONDUCTING DEVICES”, GO1K 13/006 (2013.01) ; GOIK 5/28 (2013.01) ; GOIK 5/04 (2013.01)
Technology Strategy Statement
We aim to develop a superconducting cable that can transport enough electrical current without any loss, securing sure profitability in the electricity market. To achieve this, the roadmap is divided into several phases, as shown in the high-level roadmap chart below. The first phase is to develop high-temperature superconducting materials that are mechanically strong enough to be made into long cables by 2025. The second phase, the most budget-invested phase, is to design and test a cost-effective cryogenic cooling system to maintain cables at the temperatures for the superconducting phenomenon by 2030. The third phase is to engineer and establish the technologies for some cable joints and quenching subsystems to make our roadmap technology much practicable in the market. Once the third phase is completed, our primary goal as described above is achieved. The final phase, to find and develop a room-temperature superconducting material layer, is a disruptive innovation in this field since they can eliminate the cooling system, resulting in drastic cost reduction.
Note that the graphics implemented in the arrow chart are made by DALLE-3.