Messenger RNA Vaccine
Roadmap Overview
This roadmap gives a structured overview of the mRNA vaccine technology, breaking down each phase into specific processes and tasks. Each step is crucial to ensuring the safety, efficacy, and availability of the vaccine to the masses.
Vaccine Development Technologies (1VDT)
- 2LAV: Live-attenuated vaccines
- 2IAV: Inactivated vaccines
- 2SUV: Subunit vaccines
- 2TXV: Toxoid vaccines
- 2VVV: Viral vector vaccines
- 2MRV: Messenger RNA (mRNA) vaccine
Discovery (3DCY)
The initial phase focuses on identifying and understanding the genetic composition of the target virus and creating a blueprint for the mRNA vaccine.
- 4SVI: Structural inference from viral nucleotide information
- 4MRD: mRNA design
- 4MRS: mRNA Synthesis
- 4MRM: mRNA modification
- 4LMS: Lipid Membrane Synthesis
Development (3DVT)
This stage involves testing the designed vaccine in human trials and ensuring its safety and efficacy.
- 4RMH: Risk Management of the Human Body
- 4CTM: Clinical Trial Management
Manufacturing Process (3MFP)
Once approved, the vaccine goes into mass production, ensuring that each component is assembled accurately and in the required quantities.
- 4MMS: mRNA mass synthesis (scale-up)
- 4MLS: Massive lipid synthesis
- 4MSB: Mass synthesis of buffers and other excipients
- 4MVA: Mass vaccine assembly
Actual Vaccination Situation
This final stage involves the distribution and administration of the vaccine to individuals, ensuring that they gain immunity. (Note: No specific sub-processes are provided for this step)
Design Structure Matrix (DSM) Allocation
The DSM illustrates the interplay between the tools and methods that constitute the Messenger RNA Vaccine. A 'X' sign denotes that the techniques are interconnected.
Roadmap Model using OPM
Discovery
The discovery process spans from obtaining the genetic information of the target virus to the phase just before the commencement of human clinical trials.
Development
The development process begins with the first human clinical trial and continues until the drug receives approval.
Manufacturing process
The manufacturing process begins after the drug has been approved. It starts with the assembly of each ingredient component and continues through to the shipment of the finished vaccine products.
Actual Vaccination Situation
The Actual Vaccination Situation refers to the process that starts with receiving the vaccine products at the vaccination site and culminates in individuals gaining immunity after being administered the vaccines.
Figures of Merit
Vaccine Innovations
Below is a history of vaccination innovations. While we don't have vaccines for certain diseases, the chart highlights the year in which the causative agent was linked to the disease and the year the vaccine received licensing in the US for various diseases.
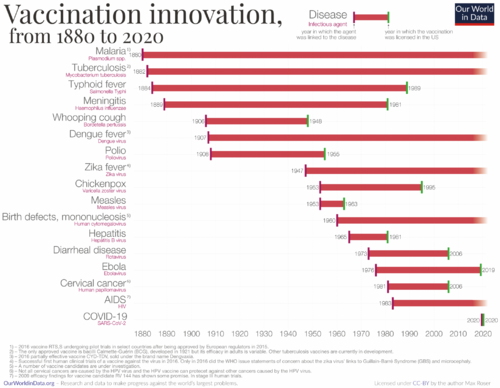
Based on the graph above, we have constructed the table below. This table indicates the duration taken for vaccine development. For the years, we've calculated the midpoint between the year the causative agent was linked to the disease and the year the vaccine received licensing in the US.
From the table above, we created the graph below.
In the realm of vaccine development, different vaccines target various pathogens or conditions. It's not accurate to assume that technological advancements will necessarily shorten the development timeframe. A significant portion of the development process is the clinical trial phase, which has become lengthier in recent times. This is due to heightened awareness and stricter requirements for drug safety. Therefore, when assessing technological advancements in this field, we must also consider other Factors of Merit (FOMs) such as the drug's effectiveness and safety profile. However, even taking these into account, progress in drug development isn't always straightforward. External influences, like regulatory standards for medicines and the inherent challenges of targeting diverse diseases, can introduce significant variability in development timelines.
Next, we focused our analysis on the FOM by examining solely the vaccine development for coronaviruses. Specifically, we compared the vaccine development processes for SARS, MERS, and COVID-19(SARS-CoV-2).
COVID-19
Discovery: Jan 11, 2020: nCov sequence released. Jan 14: VRC and Moderna Collaborate on COVID-19 vaccine design. Mar 16: 1st Phase 1 trial of USG-supported COVID-19 vaccine begins enrolling (Moderna mRNA).
Development: Nov 16, Moderna announces results of interim analysis from phase 3 study
SARS
SARS was recognized as an emerging infectious disease in March 2003.
DNA vaccine: the study from December 13, 2004 through May 2, 2005
Inactivated SARS-CoV vaccine: May to October 2004, 36 healthy people from the Beijing metropolitan area were enrolled into aPhase I clinical trial
MERS
MERS was recognized as an emerging infectious disease in September 2012.Only three potential MERS-CoV vaccine candidates have progressed to phase I clinical trials: a DNA vaccine (GLS-5300) and two viral vector-based vaccines (MVA-MERS-S and MERS001).
GLS-5300: Study Start (Actual) 2016-02.GLS-5300 is a DNA plasmid vaccine that expresses the MERS CoV spike (S) glycoprotein
MVA-MERS-S: Study Start (Actual) 2017-11-28. The vaccine contains a Modified Vaccinia Virus Ankara (MVA) vector expressing the MERS-CoV spike glycoprotein (S).
MERS001: Study Start (Actual) 2018-03-14
According to the figure above, we created the graph below.
Firstly, the COVID-19 vaccines represent the only successfully developed vaccines against coronaviruses. Their accelerated development can be attributed to prior experiences with SARS and MERS. Interestingly, the development period for the MERS vaccine was longer than that for SARS, and we aim to investigate the reasons behind this extended timeline. One potential explanation could be the challenges associated with the MERS immunological response. The rapid development of COVID-19 vaccines is linked to the research history of SARS and MERS. As illustrated in the figure below, there is a significant similarity among SARS-CoV, MERS-CoV, and SARS-CoV-2. This similarity enabled the developers of the SARS-CoV-2 vaccines to quickly identify the sequence that produces the S protein, which is the primary target of the vaccines.
Alignment with Company Strategic Drivers
Number | Strategic Drivers | Alignment targets |
---|---|---|
1 | To develop an mRNA vaccine platform that can flexibly address various diseases simply by reconfiguring the nucleotide sequence of mRNA, thereby enhancing manufacturing efficiency in terms of development duration and cost | This roadmap aims for a development period of 300 days or less for any novel pathogen. This driver is currently aligned with the 2MRV technology roadmap |
2 | To make the technical conditions required during transportation easier in order to enable the delivery of the vaccine to a broader range of regions | This roadmap aims for a recommended storage temperature of 25°C (room temperature). This driver is currently aligned with the 2MRV technology roadmap |
3 | To extend the duration of vaccine efficacy to gain a competitive edge in the market, while considering the balance between re-vaccination frequency and long-term revenue | This roadmap aims for higher efficacy, but does not set a target for duration of efficacy. This driver is not partially aligned with 2MRV. Also, a longer duration of efficacy may reduce the need for re-vaccination, potentially decreasing long-term revenue opportunities. |
Positioning of Company vs. Competition
XXX
Technical Model
XXX
Keys Publications and Patent
mRNA modification
Publications
Karikó, K., Buckstein, M., Ni, H. and Weissman, D. Suppression of RNA Recognition by Toll-like Receptors: The impact of nucleoside modification and the evolutionary origin of RNA. Immunity 23, 165–175 (2005).
First The research by Karikó et al. delves into the role of Toll-like receptors (TLRs) in recognizing RNA molecules and how modifications in RNA nucleosides can impact this recognition. TLRs are a type of protein that plays a crucial role in the innate immune system by detecting foreign molecules, including microbial pathogens. When activated, TLRs can initiate a host's response to infections, triggering potent innate immune responses that lead to subsequent adaptive immune responses.
In the context of vaccine development:
Adjuvants and Vaccine Potency: TLRs are being explored as targets for the development of vaccine adjuvants. Adjuvants are substances added to vaccines to enhance the body's immune response to the vaccine. TLR agonists, which are molecules that can activate TLRs, have been formulated in licensed vaccines to boost their efficacy. For instance, TLR agonists like monophosphoryl lipid A (MPL) and CpG-1018 are used in licensed vaccines for their adjuvant activity. Reference: Jing-Xing Yang, Jen-Chih Tseng, Guann-Yi Yu, Yunping Luo, Chi-Ying F. Huang, Yi-Ren Hong, Tsung-Hsien Chuang. "Recent Advances in the Development of Toll-like Receptor Agonist-Based Vaccine Adjuvants for Infectious Diseases".
Cancer Immunotherapy: Beyond infectious diseases, TLRs are also being explored in the realm of cancer immunotherapy. The activation of TLRs can promote both innate inflammatory responses and the induction of adaptive immunity, making them potential targets for cancer treatment. Some research has focused on developing nanoparticles that can selectively target endosomal TLRs to potentiate robust cancer vaccination. Reference: Heming Xia, Mengmeng Qin, Zenghui Wang, Yaoqi Wang, Binlong Chen, Fangjie Wan, Mingmei Tang, Xingquan Pan, Ye Yang, Jianxiong Liu, Ruiyang Zhao, Qiang Zhang, Yiguang Wang. "A pH-/Enzyme-Responsive Nanoparticle Selectively Targets Endosomal Toll-like Receptors to Potentiate Robust Cancer Vaccination".
Selective Immune Response: The study by Karikó et al. suggests that the innate immune system might detect unmodified RNA to selectively respond to bacterial infections or necrotic tissue. This selective response can be harnessed to develop vaccines that elicit a targeted immune reaction against specific pathogens.
In summary, the understanding of TLRs, their activation by RNA, and the impact of nucleoside modifications can provide valuable insights for the development of more effective vaccines and immunotherapies. The work by Karikó and others in this field lays the foundation for innovations in vaccine design and the broader field of immunology.